最新资讯
-
电话: 400-113-6988 邮箱: dongfangxicao@163.com 地址: 深圳市南山区粤海街道高新中三道9号环球数码大厦19楼
↓ 微信扫码识别关注我们 ↓
硒、硒蛋白和女性生育(综述)
发表于:2019-05-13 作者:admin 来源:本站 点击量:8779
摘要:硒(Se)是一种对动物和人类健康有着多种重要功能的必需微量元素。根据最近三大生命领域的报道,硒的生物功能往往通过硒蛋白(由人体25种基因和小鼠24种基因编码)实现。作为硒蛋白的组成部分,硒能发挥结构功能和酶活性,在后者中,其酶促和抗氧化活性已广为人知。在本综述中,我们重点关注了硒和硒蛋白的生物功能,并对硒与女性生殖功能之间的关系作了详细综述。由于此类研究大多关注于硒在妊娠中的作用,硒水平与女性生殖能力相关的数据目前仍然缺乏,只有最近硒在卵巢生理分泌作用方面的一些数据。我们对硒或硒蛋白在调节女性生殖方面的分子和生物化学机制仍所知甚少,其在人类妊娠及其并发症方面的作用仍然未被充分阐明。在低硒水平人群中进行的随机对照干预试验将会为阐明硒及硒蛋白的作用做出重要贡献。同时,对补充硒和硒蛋白(如GPX1、SELENOP和SELENOS)在卵巢功能和整体女性生殖能力方面的潜在效应的阐明也具有极大价值。
关键词:女性生育;繁殖;GPX1;不孕;卵巢功能;氧化应激;子痫前期;硒;硒蛋白
1. 引言
硒(Se)是一种在分子和组织水平上对动物和人体健康具有多种重要功能的必需微量元素,与多种病理生理学相关[1]。硒的生物功能主要通过硒蛋白(由25种人类基因和24种小鼠基因编码的含硒蛋白)形式来实现,报道显示,真核生物、古细菌、真细菌这三个生命领域都存在硒蛋白,此外,在病毒中也发现了硒蛋白的存在[2-4]。作为硒蛋白的组成成分,硒具有结构功能和酶相关功能。在后者中硒的催化和抗氧化活性被广泛认知[1]。
所有发现的哺乳动物的硒蛋白中,只有少数硒蛋白被发现具有功能活性[4]。虽然这些硒蛋白作用的分子通路和所具有重要生物功能都各有不同,但它们至少都含有一种硒代半胱氨酸(Sec,一种含硒氨基酸,它是第21种自然形成的氨基酸,与其它翻译后修饰的氨基酸不同,硒代氨基酸能通过生物合成嵌入到蛋白质中[3,5]),并且其中大部分都能发挥氧化还原酶的作用[4,6]。包括DNA的脱氧核糖核苷合成、清除有害过氧化物、被氧化的蛋白和细胞膜的还原、调控氧化还原信号、甲状腺激素代谢、蛋白质折叠和硒的运输和贮存在内的多种重要细胞生理活动均被发现需依赖于硒蛋白进行[4]。在此,我们选择了一些与繁育、生殖和发育相关的哺乳动物硒蛋白,并简述总结于表1中。
硒通常以有机硒或无机硒的形式被摄取。硒形态对其在健康和组织生长方面的潜在益处和或毒性效应有重要影响[7,40,41]。因此,对植源和动物源食物中的硒形态的考量是十分重要的,此外,我们还需要对硒被植物吸收,以及在动物体内代谢的相关生物机制进行阐明[42]。近期研究发现,膳食硒的总摄入量和硒形态均对健康效应有着重要影响,不过,硒形态对健康效应的影响可能会相对更大[42]。不同哺乳动物的硒推荐摄入量可见表2。
一些哺乳动物研究验证了硒水平与男性[52,53]、女性[53,54]生殖功能之间的关系。现有证据表明,硒在多种不良妊娠健康情况中具有重要意义,如子痫前期,自身免疫性甲状腺疾病、流产和早产[29]。此外,缺硒或许对生育率下降、胎盘滞留和生殖疾病的发生(如子宫炎)具有重要影响[55]。硒增加带来的生育率的增加或可归因于妊娠30天内的胚胎死亡率的下降[55]。大多数的女性研究主要集中在硒在妊娠中的作用[56-60],但最近也有关于硒在卵母细胞的发展和卵巢生理方面的潜能的相关研究被报道[8,53,61]。
2.硒和硒蛋白的运输
研究显示,缺硒会导致硒蛋白浓度的下降[62]。Bosl及其同事发现,纯合子Trsp(-/-)胚胎合成硒蛋白的能力低下促进了其胚胎死亡[63]。硒蛋白合成由tRNASec介导,然而tRNASec自身的数量并不太可能限制硒蛋白合成,反而硒自身的可用性可能发挥了重要作用[63]。因此,孕妇为胚胎或胎儿提供硒对硒蛋白的合成是必需的[14]。硒经胎盘进行的运输具有双向性,或会影响硒在孕妇、胎儿和新生儿组织中的净滞留[7,64]。硒是否容易穿过女性胎盘组织或渗透包含在胎盘组织中目前仍不太清楚[7,65]。小鼠血浆中的两种细胞外硒蛋白(硒蛋白P和谷胱甘肽过氧化物酶3(Gpx3))含有97%的硒[14,66]。硒蛋白P主要在肝脏中合成,再将硒运往其它大部分组织[14,67],然而关于Gpx3在硒运输方面的作用的信息却相对缺乏[14]。硒蛋白P的运输由载脂蛋白E受体2所介导,后者可作为体循环中的硒发生细胞內吞作用的媒介[14]。与其它组织和器官中的apoER2类似,胎盘中的apoER2似乎也能摄入硒蛋白P[14]。最近有报道表明硒蛋白P和Gpx3都可能参与了硒从孕妇到发育中的胚胎组织器官中的转运[14]。这些发现为硒的转运机制(卵黄囊与胎盘机制)提供了思路[14]。
小鼠实验发现,胚胎卵黄囊介导的母-胎硒转运发生在妊娠早期,晚期则通过胎盘进行[14,68]。胚胎卵黄囊能吸收子宫中含有硒蛋白P和Gpx3的液体进而实现硒运输,而胎盘则通过apoER2介导的细胞內吞作用吸收母体血液中的硒蛋白P[14]。这两种吸收机制都依赖于硒蛋白并需在特殊机体环境下进行(如缺硒)[14]。然而,也有研究表明硒的这种运输也可独立于硒蛋白P和Gpx3(血浆硒蛋白)进行[14]。硒蛋白P和Gpx3基因敲除小鼠能够在高硒饮食中存活,表明硒到组织还有其它运输途径[14,66,68]。由此可推断或许存在两种特定水平下的硒转运机制:低级机制和高级机制[14]。低级机制(可能涉及硒多糖或其它小分子硒化物)似乎并不直接依赖于硒蛋白[14],而高度依赖于妊娠母体的硒水平,这种机制降低了缺硒条件下的转运效率和在硒充足条件下的有效性 [14,68]。另一方面,高级机制(硒蛋白P-apoER2机制[69])即使在膳食硒不足的情况下也能够根据需求将硒运输到靶向组织中[14]。Burk等人发现胚胎卵黄囊介导的硒运输就具有高等运输机制的特点[14]。虽然这种运输不由硒蛋白受体介导,但它明显依赖于细胞对硒蛋白摄入,并在硒缺乏条件下也依然有效[14]。因此,无论是胚胎卵黄囊还是胎盘都能通过高等机制来实现母-胎硒运输(硒蛋白依赖),从而为缺硒孕妇的胎儿提供保护[14,68]。
3.硒蛋白在女性生殖器官中的表达
无机硒和有机硒均与硒蛋白P的表达调控相关[7]。研究发现,妊娠期大鼠的胎盘中的硒含量和硒蛋白的表达水平会同时上升[7]。许多硒蛋白如硒蛋白P、碘甲状腺原氨酸脱碘酶3(Dio3)和硫氧还蛋白还原酶(Txnrd)均会在子宫内表达[7,70]。同时,有证据发现GPX1、GPX2、GPX3、GPX4、SELENOS(也被称为VIMP)、SELENOT、SELENOF、SELENOH、SEPHS2、DIO3、DIO1和SELENOM等其它硒蛋白在牛卵巢颗粒细胞中也会表达[8]。小的、闭塞的牛卵泡中观察到了健康牛卵泡所没有的硒蛋白P表达上调和GPX1、GPX3表达下调[71]。在妊娠期小鼠中还观察到了胎盘和子宫中硒蛋白P的表达改变[7,72]。足月小鼠生产前四天其硒蛋白水平出现了不寻常地增长,并达到了峰值[72]。此外,硒蛋白在小鼠胎儿肝脏中也被发现具有早期表达[72]。
甲状腺激素对哺乳动物的生育能力尤为重要,贯穿了胚胎发育始终[73]。在人类和啮齿动物中,缺乏甲状腺激素与生育能力降低、发情周期失调、子宫结构与着床受损,以及其它哺乳动物妊娠问题相关[73]。Galton及其同事发现,妊娠小鼠的子宫中的Dio3 mRNA具有显著更高水平的表达[30]。Dio3的表达具有时间和区域特异性,例如,妊娠第九天发现子宫中层和抗中层蜕膜中存在Dio3的高度表达[7,30]。此外,着床位置也可观察到Dio3 mRNA及其相关活动[30]。Dio3在着床位置上的这种高水平表达可能是出于对暴露于母体甲状腺激素中的胎儿发育的一种保护[7,30]。此外,在人类胎盘细胞中,Dio3活性随胎龄增加而增加[7,74]。有力证据表明,妊娠期的胎盘组织在调节胎儿在母体甲状腺激素中的暴露水平方面发挥着重要作用[30]。此外,在合胞体滋养层和细胞滋养层、胎盘血管内皮层和脐带羊膜、子宫蜕膜、胎儿上皮和非妊娠子宫内膜中也发现了较高的Dio3表达[31]。这些研究结果表明,甲状腺激素水平的区域性调控对女性生育的全部阶段都具有重要作用[31]。其它一些因素也被认为对胎盘和子宫具有影响[7]。硫氧还蛋白还原酶(TPX)和TXNRD在人类和啮齿动物胎盘中也具有区域特异性表达[7,75,76]。组织化学观察表明,这两种蛋白酶都集中在子宫内膜和干细胞间质细胞的胚胎滋养层和上皮组织中[7,75,76],有研究发现,这两种蛋白能在炎症环境下保护胎盘组织。
4.硒在卵泡发育和卵巢功能中的作用
卵巢颗粒细胞的生长是卵泡发育过程(如卵泡形成)的一个重要特征。初级小滤泡的增殖(伴随着更少的卵巢颗粒细胞)发育为排卵前期的成熟卵泡(伴随着多层细胞)是卵泡生成过程中的特征性事件[77]。动物实验发现,硒也许能调控卵巢颗粒细胞的生长,成人卵巢体外实验发现,硒或许还能调控17β-雌二醇的生物合成[77]。近期研究也证实了在健康卵泡中硒和硒蛋白水平的上升,这也许是为了在卵泡后期和增殖阶段提供重要的抗氧化功能[8]。然而,人们对硒在卵巢功能和发育中的这种调节作用现在仍知之甚少[78]。Grazul-Bilska及其同事研究发现,相较于硒适当组(6μg/kg bw),孕期膳食中的高硒水平(80μg/kg bw)抑制了初级、次生、窦卵泡、基质和羊水中胎儿血管系统的生长。伴随着高硒饮食,原始卵泡增殖数和原始卵泡标记指数(增殖细胞百分比)出现下降[78]。
4.1 体外和动物模型试验证据
亚硒酸钠(无机硒)不仅能促进卵母细胞生长,还能提高膜细胞和颗粒细胞的增殖率。为了验证这一假设,Basini和Tamanini进行了一项体外实验,结果表明补充亚硒酸钠(5ng/ml)促进了牛颗粒细胞的增殖,并对E2合成起到了一定刺激作用。这些作用可以至少部分通过抑制一氧化氮的生成来实现[77]。此外,这些作用还可能受到了颗粒细胞中的某些未知的硒依赖性抗氧化酶的影响[77]。在另一项体外实验中,Kamada和Ikumo发现在培养基中添加5和200ppb的硒能促进牛黄体细胞的增殖,并具有抑制ROS危害的潜力[79]。GPX还被发现能刺激FSH的活性来抑制培养的大鼠卵巢中卵泡的凋亡[80]。另外,缺硒被证明能促进大鼠的卵巢退化和卵泡闭锁[81]。最近,Yao及其同事进行了一项体外研究来阐明硒在山羊黄体颗粒细胞增殖和甾体激素生物合成中作用的潜在机制[61]。增殖细胞核抗原(PCNA)、Akt、磷脂酰肌醇3激酶(PI3K)等生物标记物表主要在卵巢卵母细胞和颗粒细胞中表达[61]。黄体颗粒细胞增殖能被5ng/ml硒显著刺激[61]。这种增殖或许是通过上调PCNA、周期依赖激酶1(CDK1)、磷酸腺苷活化蛋白激酶(p-AMPK,Thr172)和磷酸化Akt(p-Akt,Ser473)等生物标记物的表达和下调p21的表达来实现的[61]。同样,用硒处理还促进了雌二醇分泌,并显著上调了抗氧化酶(GPX和SOD2)和甾类生成相关基因(3β-HSD和StAR)的表达[61]。此外,最近还有研究聚焦于硒在牛卵巢组织中的生物积累[8]。Ceko及其同事通过射线荧光成像技术成功在大的、健康的卵泡中鉴定出了硒,同时,与黄体相比,在牛卵泡的细胞膜上鉴定出了高出黄体10倍左右的硒含量[8]。这些发现均表明,抗氧化型的硒蛋白主要在卵泡中发挥潜在作用,例如,在升高的ROS及其相关氧化损伤中保护卵泡。
4.2 人类研究证据
女性生殖与硒水平、硒依赖性GPX催化活性之间的关系在极少数的几项研究中得到了验证,这些研究发现,低硒与低滤泡液水平与更高的不孕症发生有关[82]。1995年,Paszkowski及其同事首次在人类滤泡液中发现了硒,其中的酶活性表现出硒依赖性[10]。研究者们还评估了来自112位患者阴道卵母细胞的135个滤泡液样本,发现相较于那些知晓病因的不孕症患者(如输卵管不孕或与男性因素相关的不孕),具有特发性不孕症的患者的滤泡硒水平显著降低[10]。基于该发现,研究者们推测,滤泡微环境中的含硒酶GPX的抗氧化活性或许在配子发生和受精过程中发挥了重要作用[10]。最近,Ceko的研究团队提出GPX1在测定卵泡生长、成熟和优势中具有重要作用[8]。基于此,源自卵丘卵母细胞复合体(COCs)的人类卵丘细胞(CCs)被用于体外受精(IVF)和卵母细胞胞浆内单精子注射[8]。这些在胚胎移植前从COCs中恢复的CCs在妊娠期中的GPX1表达明显高于那些妊娠失败者[8]。许多研究者们还发现在膳食硒缺乏的情况下会出现GPX1表达下降,这表明部分卵巢疾病也许能通过补硒进行改善[8]。这些发现将会成为饮食硒摄入、GPX1的体内表达、排卵相关疾病等相关流行病学研究的基础[8,9]。
5. 硒在卵巢病理及辅助生殖技术相关的氧化应激中的意义
氧化应激、女性生育能力降低和缺硒之间的相关性是另一个需要我们调查和研究的领域[83]。一个有益的事实是,子宫内膜异位症(如多囊性子宫内膜异位症)中ROS的过度生成可能导致硒的过度消耗(因硒的抗氧化作用)。低硒水平则会导致伴随着ROS增加的氧化还原水平失衡,后者被认为是多囊卵巢综合症的诱发因素。无论如何,机体的低硒水平会导致对自由基诱导损伤的防御下降,这或许可归因于硒参与硒蛋白的形成并与其抗氧化能力密切相关[84]。
5.1 人类研究
对患有不孕症或原发性卵巢功能不全(POI)的卵巢自身免疫性患者的鉴定也许可借鉴化疗、环境、遗传和辐射相关因素患者并寻找替代治疗方案[85]。硒结合蛋白1(SBP1)对卵巢自身免疫相关的不孕症和POI具有特殊意义[85]。Edassery及其同事在特发性不孕症的妇女中发现了与血清自身抗体相关的抗原分子。在患有特发性不孕症和卵巢功能早衰的女性中发现了明显更高水平的SBP1[85]。印度的Singh等人研究了经历过体外受精(IVF)的子宫内膜异位症和输卵管不孕症的女性的滤泡液中的氧化应激标记物,结果发现与对照相比,子宫内膜异位症患者的滤泡液中的ROS和丙二醛生成显著增加[86]。此外,相较于长期输卵管不孕不育的女性,子宫内膜异位症合并不孕的女性滤泡液中的硒水平显著降低[86]。2013年土耳其的一项研究表明,36位患有多囊卵巢综合征的女性的血浆硒水平显著低于对照组(n=33,BMI健康女性)[87]。这些多囊卵巢综合征女性患者的硒水平降低可能与高雄激素血症有关[87]。
此外,适量补充包括硒在内的微量元素被发现也许能显著影响滤泡微环境中的氧化还原平衡,从而影响IVF结果[88]。最近两项关于ART的临床试验(Luddi等[88]和Jimenez Tunon等[89])发现,补硒(以微量元素形式)也许能改善IVF结局(表3)。这些研究均因其样本量过少而存在局限性,另一局限性在于这两项实验均是将硒与其它微量元素合用进行补充,因而不能完全排除其它微量元素的影响。这些发现或许能在一定程度上为临床医生应对经IVF治疗的女性时提供一些参考[88]。然而,我们仍需对孕妇在怀孕期间的营养进行的机械和流行病学研究,特别是对多种微量营养素及其相互作用的研究,来充分阐明这些因素在成功生育和怀孕中的重要性[90]。
5.2 体外研究
一般来说,胚胎因比成年人更低的抗氧化酶活性从而使其对氧化损伤尤为敏感[91,92]。为了维持胚胎的生存能力与质量,一般需要特定的培养基。虽然,许多胚胎的培养环境容易实现,但要实现胚胎发育的最优环境却具一定的困难。目前,一项关于促进囊胚发育物质的研究正在进行[93,94]。为了确保所培养的细胞中的硒蛋白能正常进行生物合成,主流培养基中往往都会添加硒元素[94-96]。具有酶促活性的GPX不仅依赖于硒和维生素B6,同时也依赖于谷胱甘肽(GSH)活性[97]。猪卵母细胞中的GSH的数量不仅与受精相关,也与促进胚胎着床发育相关。胚胎期的GSH会不断减少,并在在囊胚期达到最低点。值得注意的是,在这一时期,内源性ROS的水平将达到最高[98]。由此可推断,GSH的降低与ROS的升高对正常分化过程具有一定的功能性意义[99,100]。此外,有研究发现,ROS或许对小鼠胚泡中的细胞凋亡发挥着重要调控作用[99]。
在胚胎培养基中添加硒能减少氧化损伤、调控细胞凋亡进而提高猪单性生殖胚胎的发育能力和总体质量[94]。在培养基中添加亚硒酸钠(2.5和25ng/ml)也被发现能提高囊胚率、细胞数和内细胞群比例,从而减少猪孤雌生殖胚胎中的细胞凋亡,以及BAX/BCL-xL基因比值和Caspase3的表达,并提高其GPX和ERK1/2的表达[94]。此外,补硒还能提高体外成熟卵母细胞细胞内的GPX浓度和活性,调控基因表达,改善体外受精的牛胚胎的囊胚发育和质量。近期中国的一个研究团队研究了滤泡液中的硒浓度和补硒对细胞分裂的影响,CCs中DNA的完整性,卵母细胞的发育能力,去除卵丘的卵母细胞中的GPX活性,和体外培育的牦牛卵母细胞中的硒相关基因的表达[53]。随着在体外培养基中添加硒(2和4μg/ml亚硒酸钠),与对照组(0μg/ml亚硒酸钠)相比,加硒组中所培养的卵丘细胞中的DNA损伤显著降低。同时,总GPX活性、囊胚形成率以及硒相关基因的表达都被显著上调[53]。
另一项体外研究聚焦于亚硒酸钠的作用。Abedelahi的实验组研究发现,在培养基中添加5或10ng/ml亚硒酸钠能减轻从玻璃化和非玻璃化卵巢组织中获得的窦前卵泡中由ROS引起的氧化应激,并提高其总抗氧化能力(TAC)和GPX活性[101]。此外,硒处理组的卵泡、卵母细胞和胚胎的发育率也显著上升[101]。同样的,在其早期的体外研究中,该研究团队也发现在培养基中添加5和10ng/ml亚硒酸钠将会对卵泡和卵母细胞的生长和生存能力发挥出出剂量依赖性的改善作用,这些作用或可归因于硒能以剂量依赖的方式发挥广谱抗氧化能力[102]。综上所述,这些发现可在某种程度上为促进体外卵母细胞成熟以及提高哺乳动物卵母细胞和胚胎的发育能力提供一些参考,也可作为该领域未来研究的基础。
6.母体补充膳食硒的作用
在妊娠期间,母体补充膳食硒不仅能加强抗氧化活性,促进雌二醇、孕酮和T4生成,还能提高机体对主要营养成分的新陈代谢[103]。最近几项研究评估了使用有机硒或无机硒合并其它维生素(如VB6)对母体进行营养补充的作用。结果表明,VB6对有机硒在硒依赖性GPX的抗氧化防御系统中的代谢有着重要作用[104-106]。补充膳食硒对母体的氧化应激、抗氧化活性、胚胎发育和生育能力的影响相关的一系列动物实验被总结在表4中。
7.硒和胎盘中的氧化应激
补硒对GPX和TXNRD等内源性抗氧化酶的表达和活性是非常重要的。在细胞实验中,补硒缓解了细胞模型中的氧化应激引发的损伤,从而为微量元素补充剂在减轻某些妊娠相关疾病中的安全效用和关键机制提供了些许思路[32,110-112]。胎盘中的氧化应激对妊娠并发症(如子痫前期,子宫内生长受限,妊娠期糖尿病和早产)的病理生理和发生具有重要意义[110]。充分阐明胎盘氧化应激引起的胚胎滋养层细胞凋亡的相关机制也许能为先兆子痫的预防提供新的干预措施,据报道,先兆子痫为美国带来了每年超过8万的早产儿,在全球范围内造成了每年约七万五千名产妇的死亡[19,111]。近期一些报道揭示了硒提高整体滋养层细胞的线粒体功能和生物合成的潜在机制[32,59,110-112]。这些结果表明,硒带来的这些益处是通过提高抗氧化功能、减少ROS产物从而保护线粒体功能并增加线粒体生物合成来实现的[112]。此外,SELENOH被发现能激活NRF1和PGC-1α等转录因子来促进胚胎滋养层细胞中的线粒体生物合成[113]。这些发现有助于阐明滋养层细胞对氧化应激的响应机制,以及硒如何调控相关基因,进而通过调节线粒体功能来改善细胞存活率及其侵袭能力[114]。补硒在改善胎盘滋养层细胞系的线粒体生物合成和功能的近期相关研究汇总于表5中。
8.硒对妊娠的影响
大量研究已经证实,硒参与了GPX、SELENOP、TXNRD族等硒蛋白的生物合成,从而对妊娠有着重要作用,尤其是在妊娠期间氧化应激增加的情况下[115]。因此,抗氧化防御机制对调控氧化应激极其重要,甚至还与围产期发病率和死亡率相关[116-118]。值得注意的是,硒水平与妊娠并发症进展的关系现在仍未被充分阐明。然而,低硒水平与不良胚胎结局之间的关系已被证实。大量横截面和病例对照实验并未发现两者之间存在明显的因果关系。因此我们可以推断,妊娠并发症及随后结果可能与低硒水平有关,也可能仅仅是母体对氧化应激状态升高的简单生理反应[119]。
“妊娠前硒水平是否比妊娠期硒水平对预防妊娠高血压更重要?”这一假设目前仍需进一步验证[15]。一般来说,硒水平可能会对卵母细胞发育、受精以及着床带来影响[8,15]。因此推荐的做法是在妊娠期增加硒摄入[120]。此外,一些关于硒对生殖健康作用的研究推荐的妊娠期最佳补硒时间点差异较大。因此,迄今为止补硒的最佳时间依然没有定论[121]。与硒相关的关键信息缺乏,在很大程度上得到了其他报告的补充,这些报告鼓励进一步阐明补充微量元素的时间点对妊娠结果的潜在影响[122,123]。2017年,Mamon和Ramos进行的一项小鼠研究指出,妊娠期间补硒(3.0μg/d)的最佳时间在于围孕期的不同阶段[121]。与对照组及妊娠前-妊娠期补硒组相比,研究者们仅在妊娠前补硒组和仅在妊娠期补硒组中观察到了囊胚质量的提高和胚胎植入前的损失降低[121]。这些结果表明,妊娠前和妊娠期是围孕期补硒的两个最佳时间段,仅在其中一个时期补硒能提高囊胚的发育能力和胚胎植入的成功率[121]。
最近,一项小样本实验表明,硒与多种妊娠并发症相关[15,37,124,125]。研究发现,基线硒水平的人群的血清硒与SELENOP水平正相关[124]。此外,在正常怀孕期间,全血硒浓度因妊娠发生下降了12%[37,126,127]。血浆体积相同的情况下,妊娠期全血硒浓度下降的另一可能原因在于硒通过在胎盘中高度表达的SELENOP被转运给了胎儿[68,72]。相反,更高的硒水平则与更低风险的流产和早产相关。一些随机对照试验表明,高硒摄入或高硒水平对子痫前症和自身免疫性甲状腺疾病有益。硒能改善氧化应激、内质网应激和炎症(图1),从而保护内质网,调控类二十烷酸的生成,调控血管张力,以及抑制感染,这些对妊娠期可能都是至关重要的[29]。
英国的一项覆盖了230名初产孕妇的双盲安慰剂对照先导实验中,将妊娠12-14周的孕妇随机分组并为其提供60μg/d富硒酵母或安慰剂直至分娩[37]。结果发现,补硒组在12-35周之间的全血硒浓度显著增加,而安慰剂组则显著降低。同样的,补硒组在妊娠35周时的全血硒和血浆SELENOP水平显著高于安慰剂组[37]。此外,该研究还发现补硒和血清可溶性血管内皮生长因子受体-1(sFlt-1)呈负相关,表明补硒有益于限制sFlt-1的生成从而具有抗血管生成的能力,并有益于改善子痫前期的病理生理。这是唯一调查并得出了“低硒水平人群补硒与子痫前期风险相关”结果的研究[37]。此外,最近一项报告显示波兰孕妇孕周硒水平逐月下降,导致大量孕妇发展成严重缺硒。妊娠期女性的平均硒和SELENOP水平也被发现在不断下降[124]。该研究发现在所有组的孕妇的三个月妊娠期中,硒与SELENOP浓度正相关[124]。相同现象出现在最近另一项关于波兰母亲与子女定群的研究中:孕妇的血浆硒浓度从第一个月到第三个月下降了23%(48.3+/-10.6到37.3+/-9.8μg/L)[125]。
子宫内生长受限是围产期死亡率的首要原因,影响了10-15%孕妇[128,129]。有报道显示,更高水平的ROS和炎症生物标记物在子宫内生长限制的发育和病理过程中起重要作用[130,131]。Mesdaghinia及其同事开展的一项随机双盲安慰剂对照试验以研究补硒对子宫内生长限制高风险孕妇(n=60)的临床症状和代谢状况的影响[131]。
硒还与其它妊娠并发症相关,如习惯性流产[56,132,133]、小于胎龄儿[119,134]和产后甲状腺功能不足[135]。一些随机对照试验和观察性研究发现,硒能减少甲状腺过氧化物酶抗体水平以及甲状腺功能减退和产后甲状腺疾病的发生[136]。甲状腺过氧化物酶抗体阳性的孕妇将会有更高风险发展成产后甲状腺功能不全(PPTD)和永久性甲状腺功能减退[135]。而硒被发现能减轻自身免疫性甲状腺炎患者的甲状腺炎[135]。一项关于甲状腺过氧化物酶抗体阳性(TPOAb阳性)孕妇的高质量随机对照试验[135]发现,与安慰剂对照组相比,每天补充200μg硒代蛋氨酸显著减少了TPOAb效价,降低了甲状腺炎、产后甲状腺疾病和永久性甲状腺功能减退的发生[135]。虽然现有文献证明补硒能以某种方式有益于减少缺硒孕妇并发症发生,然而,正在开展或未来将要开展的干预实验结果可能会加强或否定增加硒摄入的观点。
9.硒和硒蛋白对生殖系统癌症的影响
人们关于硒在癌症发生和癌症治疗方面的作用已经争论了数十年,硒蛋白会预防、抑制还是促进癌症发展目前仍争论不休[137]。卵巢癌是女性第五大癌症死因,具有最高的总体死亡率和较低的五年生存率[138]。超过90%的卵巢癌是上皮性卵巢癌(EOC),EOC中包括了病理和分子学特征不同的多种类型[138]。一些文献证据表明,硒和硒蛋白能在卵巢癌等女性生殖系统癌症中发挥作用 [139]。与健康女性相比,患有宫颈良性肿瘤及癌症、子宫体癌或卵巢癌的女性的血浆硒和GPX活性显著降低[140]。这些发现表明,患有女性生殖系统相关肿瘤的女性往往伴随着更低的抗氧化能力(与硒相关)[140]。Agnani等发现,患有乳头状浆液性卵巢癌的女性的GPX3活性呈阶段依赖性降低[139]。同时,在肿瘤复发的女性患者中的血浆GPX3水平也被观察到出现降低,且当患者和对照组只纳入50岁以上的女性时,这些水平依赖性降低变得更加明显[139]。此外,在大鼠和人类中观察到在所有级别的子宫内膜腺癌中均出现了GPX3下调[139,141]。一些早期研究发现,细胞上皮性卵巢癌组织中的GPX3高于对照组[142-144]。虽然其潜在分子学机制未被充分阐明,但这些结果已经表明GPX3活性存在肿瘤特异性[138]。在一项近期研究中,Wu及其同事发现六氟化铂敏感组中的GPX3相较于六氟化铂耐受组被下调[138]。故此可推断,GPX3抑制肿瘤的作用机制可能主要归因于启动子的超甲基化、c-Met表达的失调以及能清除有害自由基的抗氧化酶的作用[138]。然而,GPX3响应抗肿瘤药物的这种潜在机制中仍然存在大量未知,需要进一步阐明[138]。不过,硒和硒蛋白的这些作用也许能为生殖系统癌症的靶向治疗和预防提供策略。
10.总结
在本综述中,我们综合阐述了硒与硒蛋白在生殖以及一些与动物、人类生育相关疾病中的作用。从早期动物和人类研究中可看出,硒与硒蛋白能调控抗氧化平衡,因而对女性的最优生殖而言是必需的。
硒与硒蛋白在某些女性生育领域的作用目前仍未被充分阐明,需要进一步研究与思考,这些领域包括母体膳食硒摄入对氧化应激的影响,硒对胎儿卵巢发育与功能的影响,补硒对改善胎盘氧化应激的作用等。此外,人们对怀孕前时期的卵巢生理学方面,尤其是激素合成和卵巢滤泡发育方面关注较少,此外,生殖效率和生殖健康也缺乏关注。最近一些动物体外实验证据表明硒和硒蛋白在卵母细胞成熟和受精中具有潜在作用,然而体内对照试验仍然大幅度缺乏。此外,硒与硒蛋白在不同卵巢疾病(包括癌症)中作用的证据也较少且具有较大不确定性。同时,硒和硒蛋白在改善由辅助生殖技术(如IVF)和胚胎发育引起的氧化和氮化应激中的作用也未被充分阐明。另外,硒和硒蛋白与女性生殖(动物和人类)之间的关系仍存在许多问题等待人们回答。对这些问题的回答将会有助于阐明硒这种潜力非凡的微量元素对女性生育及健康的潜在生物功能。
补硒的响应曲线呈现U型,这意味着补硒虽然对缺硒个体有益,但对硒含量充足的个体或许会为其带来健康风险[145]。因此,我们必须将硒摄入量维持在合理范围,特别是对那些具有适当或高硒水平的人群。关于补硒对妊娠期的影响,一些在硒水平较低的人群中进行的高质量随机对照试验呈现出了令人振奋的结果[37]。然而,这些试验在未来仍需要纳入更多样本数以阐明硒和硒蛋白减少妊娠相关并发症(如先兆子痫)风险的潜在分子和生物化学机制。在本综述中,目前正在进行的实验和荟萃分析应该很快就能得出适当的建议。目前,向孕妇销售的含硒补充剂的使用量很大,而在发达国家,孕妇食用此类补充剂的比例非常高,这使得研究者们越来越难将受试者(孕妇)纳入随机对照试验。
综上所述,关于硒和硒蛋白在女性生殖中的改善作用的现有证据水平和质量都很高,但是这类研究的数量还不足以得出可靠的结论。因此,阐明补充硒和含硒蛋白(如GPX、SELENOP、SELENOS)对卵巢功能、妊娠相关并发症和女性整体生殖能力的潜在影响将具有巨大价值。
原文:Qazi I, Angel C, Yang H, et al. Selenium, Selenoproteins, and Female Reproduction: A Review[J]. Molecules, 2018, 23(12): 3053.
References
1. Rayman, M.P. The importance of selenium to human health. The Lancet 2000, 356, 233–241. [CrossRef]
2. Labunskyy, V.M.; Hatfield, D.L.; Gladyshev, V.N. Selenoproteins: molecular pathways and physiological roles. Physiol. Rev. 2014, 94, 739–777. [CrossRef] [PubMed]
3. Kryukov, G.V.; Castellano, S.; Novoselov, S.V.; Lobanov, A.V.; Zehtab, O.; Guigó, R.; Gladyshev, V.N. Characterization of mammalian selenoproteomes. Science 2003, 300, 1439–1443. [CrossRef] [PubMed]
4. Papp, L.V.; Lu, J.; Holmgren, A.; Khanna, K.K. From selenium to selenoproteins: synthesis, identity, and their role in human health. Antioxid. Redox Signaling 2007, 9, 775–806. [CrossRef] [PubMed]
5. Gladyshev, V.N.; Arnér, E.S.; Berry, M.J.; Brigelius-Flohé, R.; Bruford, E.A.; Burk, R.F.; Carlson, B.A.; Castellano, S.; Chavatte, L.; Conrad, M. Selenoprotein gene nomenclature. J. Biol. Chem. 2016, 291,
24036–24040. [CrossRef] [PubMed]
6. Zoidis, E.; Seremelis, I.; Kontopoulos, N.; Danezis, G.P. Selenium-Dependent Antioxidant Enzymes: Actions and Properties of Selenoproteins. Antioxidants 2018, 7, 66. [CrossRef] [PubMed] Molecules 2018, 23, 3053 18 of 24
7. Pappas, A.; Zoidis, E.; Surai, P.; Zervas, G. Selenoproteins and maternal nutrition. Comp. Biochem. Physiol. Part. B: Biochem. Mol. Biol. 2008, 151, 361–372. [CrossRef] [PubMed]
8. Ceko, M.J.; Hummitzsch, K.; Hatzirodos, N.; Bonner,W.M.; Aitken, J.B.; Russell, D.L.; Lane, M.; Rodgers, R.J.; Harris, H.H. X-Ray fluorescence imaging and other analyses identify selenium and GPX1 as important in female reproductive function. Metallomics 2015, 7, 71–82. [CrossRef] [PubMed]
9. Ceko, M.J.; Hummitzsch, K.; Bonner,W.M.; Aitken, J.B.; Spiers, K.M.; Rodgers, R.J.; Harris, H.H. Localization of the trace elements iron, zinc and selenium in relation to anatomical structures in bovine ovaries by X-ray fluorescence imaging. Microsc. Microanal. 2015, 21, 695–705. [CrossRef] [PubMed]
10. Paszkowski, T.; Traub, A.; Robinson, S.; McMaster, D. Selenium dependent glutathione peroxidase activity in human follicular fluid. Clin. Chim. Acta 1995, 236, 173–180. [CrossRef]
11. Baek, I.J.; Yon, J.M.; Lee, S.R.; Kim, M.R.; Hong, J.; Lee, B.; Yun, Y.; Nam, S.Y. Differential Expression of Gastrointestinal Glutathione Peroxidase (GI-GPx) Gene during Mouse Organogenesis. Anat. Histol. Embryo. 2011, 40, 210–218. [CrossRef] [PubMed]
12. Xu, X.; Leng, J.-Y.; Gao, F.; Zhao, Z.-A.; Deng,W.-B.; Liang, X.-H.; Zhang, Y.-J.; Zhang, Z.-R.; Li, M.; Sha, A.-G. Differential expression and anti-oxidant function of glutathione peroxidase 3 in mouse uterus during decidualization. FEBS Lett. 2014, 588, 1580–1589. [CrossRef] [PubMed]
13. Santos, C.; García-Fuentes, E.; Callejón-Leblic, B.; García-Barrera, T.; Gómez-Ariza, J.L.; Rayman, M.P.; Velasco, I. Selenium, selenoproteins and selenometabolites in mothers and babies at the time of birth. Br. J. Nutr. 2017, 117, 1304–1311. [CrossRef] [PubMed]
14. Burk, R.F.; Olson, G.E.; Hill, K.E.;Winfrey, V.P.; Motley, A.K.; Kurokawa, S. Maternal-fetal transfer of selenium in the mouse. FASEB J. 2013, 27, 3249–3256. [CrossRef] [PubMed]
15. Rayman, M.P.; Bath, S.C.;Westaway, J.;Williams, P.; Mao, J.; Vanderlelie, J.J.; Perkins, A.V.; Redman, C.W. Selenium status in UK pregnant women and its relationship with hypertensive conditions of pregnancy. Br. J. Nutr. 2015, 113, 249–258. [CrossRef] [PubMed]
16. Hayashi, K.-G.; Ushizawa, K.; Hosoe, M.; Takahashi, T. Differential genome-wide gene expression profiling of bovine largest and second-largest follicles: identification of genes associated with growth of dominant follicles. Reprod. Biol. Endocrinol. 2010, 8, 11. [CrossRef] [PubMed]
17. Yant, L.J.; Ran, Q.; Rao, L.; Van Remmen, H.; Shibatani, T.; Belter, J.G.; Motta, L.; Richardson, A.; Prolla, T.A. The selenoprotein GPX4 is essential for mouse development and protects from radiation and oxidative damage insults. Free Radical Biol. Med. 2003, 34, 496–502. [CrossRef]
18. Imai, H.; Hirao, F.; Sakamoto, T.; Sekine, K.; Mizukura, Y.; Saito, M.; Kitamoto, T.; Hayasaka, M.; Hanaoka, K.; Nakagawa, Y. Early embryonic lethality caused by targeted disruption of the mouse PHGPx gene. Biochem. Biophys. Res. Commun. 2003, 305, 278–286. [CrossRef]
19. Mistry, H.D.;Wilson, V.; Ramsay, M.M.; Symonds, M.E.; Pipkin, F.B. Reduced selenium concentrations and glutathione peroxidase activity in preeclamptic pregnancies. Hypertension 2008, 52, 881–888. [CrossRef]
[PubMed]
20. Peng, X.; Lin, Y.; Li, J.; Liu, M.;Wang, J.; Li, X.; Liu, J.; Jia, X.; Jing, Z.; Huang, Z. Evaluation of Glutathione Peroxidase 4 role in Preeclampsia. Sci. Rep. 2016, 6, 33300. [CrossRef] [PubMed]
21. Malinova, M.; Paskaleva, V. Selenium and glutathione peroxidase in patients with preeclampsia. Akush. Ginekol. 2013, 52, 3–7.
22. Imai, H.; Hakkaku, N.; Iwamoto, R.; Suzuki, J.; Suzuki, T.; Tajima, Y.; Konishi, K.; Minami, S.; Ichinose, S. Ishizaka, K. Depletion of selenoprotein GPx4 in spermatocytes causes male infertility in mice. J. Biol. Chem. 2009, 284, 32522–32532. [CrossRef] [PubMed]
23. Ahsan, U.; Kamran, Z.; Raza, I.; Ahmad, S.; Babar, W.; Riaz, M.; Iqbal, Z. Role of selenium in male reproduction—A review. Anim. Reprod. Sci. 2014, 146, 55–62. [CrossRef] [PubMed]
24. Chabory, E.; Damon, C.; Lenoir, A.; Kauselmann, G.; Kern, H.; Zevnik, B.; Garrel, C.; Saez, F.; Cadet, R.; Henry-Berger, J. Epididymis seleno-independent glutathione peroxidase 5 maintains sperm DNA integrity in mice. J. Clin. Investing. 2009, 119, 2074–2085. [CrossRef] [PubMed]
25. Jakupoglu, C.; Przemeck, G.K.; Schneider, M.; Moreno, S.G.; Mayr, N.; Hatzopoulos, A.K.; de Angelis, M.H.; Wurst,W.; Bornkamm, G.W.; Brielmeier, M. Cytoplasmic thioredoxin reductase is essential for embryogenesis but dispensable for cardiac development. Mol. Cell. Biol. 2005, 25, 1980–1988. [CrossRef] [PubMed] Molecules 2018, 23, 3053 19 of 24
26. Bondareva, A.A.; Capecchi, M.R.; Iverson, S.V.; Li, Y.; Lopez, N.I.; Lucas, O.; Merrill, G.F.; Prigge, J.R.; Siders, A.M.;Wakamiya, M. Effects of thioredoxin reductase-1 deletion on embryogenesis and transcriptome. Free Radical Biol. Med. 2007, 43, 911–923. [CrossRef] [PubMed]
27. Conrad, M.; Jakupoglu, C.; Moreno, S.G.; Lippl, S.; Banjac, A.; Schneider, M.; Beck, H.; Hatzopoulos, A.K.; Just, U.; Sinowatz, F. Essential role for mitochondrial thioredoxin reductase in hematopoiesis, heart development, and heart function. Mol. Cell. Biol. 2004, 24, 9414–9423. [CrossRef] [PubMed]
28. Su, D.; Novoselov, S.V.; Sun, Q.-A.; Moustafa, M.E.; Zhou, Y.; Oko, R.; Hatfield, D.L.; Gladyshev, V.N. Mammalian selenoprotein thioredoxin-glutathione reductase roles in disulfide bond formation and sperm maturation. J. Biol. Chem. 2005, 280, 26491–26498. [CrossRef] [PubMed]
29. Rayman, M.P. Selenium and adverse health conditions of human pregnancy. In Selenium; Springer: New York, NY, USA, 2011; pp. 531–544.
30. Galton, V.A.; Martinez, E.; Hernandez, A.; Germain, E.A.S.; Bates, J.M.; Germain, D.L.S. Pregnant rat uterus expresses high levels of the type 3 iodothyronine deiodinase. J. Clin. Investing. 1999, 103, 979–987. [CrossRef] [PubMed]
31. Huang, S.A.; Dorfman, D.M.; Genest, D.R.; Salvatore, D.; Larsen, P.R. Type 3 iodothyronine deiodinase is highly expressed in the human uteroplacental unit and in fetal epithelium. J. Clin. Endocrinol. Metab. 2003, 88, 1384–1388. [CrossRef] [PubMed]
32. Khera, A.; Vanderlelie, J.; Perkins, A. Selenium supplementation protects trophoblast cells from mitochondrial oxidative stress. Placenta 2013, 34, 594–598. [CrossRef] [PubMed]
33. Michaelis, M.; Gralla, O.; Behrends, T.; Scharpf, M.; Endermann, T.; Rijntjes, E.; Pietschmann, N.; Hollenbach, B.; Schomburg, L. Selenoprotein P in seminal fluid is a novel biomarker of sperm quality. Biochem. Biophys. Res. Commun. 2014, 443, 905–910. [CrossRef] [PubMed]
34. Renko, K.;Werner, M.; Renner-Muller, I.; Cooper, T.G.; Yeung, C.H.; Hollenbach, B.; Scharpf, M.; Kohrle, J.; Schomburg, L.; Schweizer, U. Hepatic selenoprotein P (SePP) expression restores selenium transport and prevents infertility and motor-incoordination in Sepp-knockout mice. Biochem. J. 2008, 409, 741–749. [CrossRef] [PubMed]
35. Olson, G.E.;Winfrey, V.P.; NagDas, S.K.; Hill, K.E.; Burk, R.F. Apolipoprotein E receptor-2 (ApoER2) mediates selenium uptake from selenoprotein P by the mouse testis. J. Biol. Chem. 2007, 282, 12290–12297. [CrossRef] [PubMed]
36. Olson, G.E.;Winfrey, V.P.; NagDas, S.K.; Hill, K.E.; Burk, R.F. Selenoprotein P is required for mouse sperm development. Biol. Reprod. 2005, 73, 201–211. [CrossRef] [PubMed]
37. Rayman, M.P.; Searle, E.; Kelly, L.; Johnsen, S.; Bodman-Smith, K.; Bath, S.C.; Mao, J.; Redman, C.W. Effect of selenium on markers of risk of pre-eclampsia in UK pregnant women: a randomised, controlled pilot trial. Br. J. Nutr. 2014, 112, 99–111. [CrossRef] [PubMed]
38. Moses, E.K.; Johnson, M.P.; Tommerdal, L.; Forsmo, S.; Curran, J.E.; Abraham, L.J.; Charlesworth, J.C.; Brennecke, S.P.; Blangero, J.; Austgulen, R. Genetic association of preeclampsia to the inflammatory response gene SEPS1. Am. J. Obstet. Gynecol. 2008, 198, 20. [CrossRef] [PubMed]
39. Turanov, A.A.; Malinouski, M.; Gladyshev, V.N. Selenium and male reproduction. In Selenium: Its Molecular Biology and Role in Human Health; Hatfield, D., Berry, M., Gladyshev, V., Eds.; Springer: New York, NY, USA, 2011; pp. 409–417.
40. Letavayová, L.; Vlˇcková, V.; Brozmanová, J. Selenium: from cancer prevention to DNA damage. Toxicology 2006, 227, 1–14. [CrossRef] [PubMed]
41. Schrauzer, G.N. Selenomethionine: A review of its nutritional significance, metabolism and toxicity. J. Nutr. 2000, 130, 1653–1656. [CrossRef] [PubMed]
42. Rayman, M.P.; Infante, H.G.; Sargent, M. Food-chain selenium and human health: spotlight on speciation. Br. J. Nutr. 2008, 100, 238–253. [CrossRef] [PubMed]
43. Institute of Medicine: Panel on Dietary Antioxidants and Related Compounds: Dietary Reference Intakes for Vitamin C, Vitamin E, Selenium, and Carotenoids. Washington (DC). Available online: https://www.ncbi. nlm.nih.gov/books/NBK225470/ (accessed on 19 November 2018).
44. Council, N.R. Nutrient Requirements of Small Ruminants: Sheep, Goats, Cervids, and New World Camelids; The National Academies Press: Washington, DC, USA, 2007; p. 384.
45. Surai, P.F.; Fisinin, V.I. Selenium in Pig Nutrition and reproduction: Boars and semen quality—A Review. Asian-Australas. J. Anim. Sci. 2015, 28, 730. [CrossRef] [PubMed] Molecules 2018, 23, 3053 20 of 24
46. Council, N.R. Nutrient Requirements of Horses: Sixth Revised Edition; The National Academies Press: Washington, DC, USA, 2007; p. 360.
47. Geor, R.J.; Coenen, M.; Harris, P. Equine Applied and Clinical Nutrition E-Book: Health, Welfare and Performance. FEMS Microbiol. Lett. 2014, 57, 73–77.
48. Council, N.R. Nutrient Requirements of Dairy Cattle: Seventh Revised Edition, 2001; The National Academies Press: Washington, DC, USA, 2001; p. 408.
49. Council, N.R. Nutrient Requirements of Beef Cattle: Seventh Revised Edition: Update 2000; The National Academies Press: Washington, DC, USA, 2000; p. 248.
50. Faye, B.; Seboussi, R. Selenium in camel–A review. Nutrients 2009, 1, 30–49. [CrossRef] [PubMed]
51. Rayman, M.P. The use of high-selenium yeast to raise selenium status: how does it measure up? Br. J. Nutr. 2004, 92, 557–573. [CrossRef] [PubMed]
52. Foresta, C.; Flohé, L.; Garolla, A.; Roveri, A.; Ursini, F.; Maiorino, M. Male fertility is linked to the selenoprotein phospholipid hydroperoxide glutathione peroxidase. Biol. Reprod. 2002, 67, 967–971. [CrossRef] [PubMed]
53. Xiong, X.; Lan, D.; Li, J.; Lin, Y.; Li, M. Selenium supplementation during in vitro maturation enhances meiosis and developmental capacity of yak oocytes. Anim. Sci. J. 2018, 89, 298–306. [CrossRef] [PubMed]
54. Kommisrud, E.; Østerås, O.; Vatn, T. Blood selenium associated with health and fertility in Norwegian dairy herds. Acta Vet. Scand. 2005, 46, 229. [CrossRef] [PubMed]
55. Mehdi, Y.; Dufrasne, I. Selenium in cattle: a review. Molecules 2016, 21, 545. [CrossRef] [PubMed]
56. Al-Kunani, A.; Knight, R.; Haswell, S.; Thompson, J.; Lindow, S. The selenium status of women with a history of recurrent miscarriage. BJOG: An. Int. J. Obstet. Gynaecol. 2001, 108, 1094–1097.
57. Güvenç, M.; Güven, H.; Karata¸s, F.; Aygün, A.D.; Bekta¸s, S. Low levels of selenium in miscarriage. J. Trace Elements Exp. Med. 2002, 15, 97–101. [CrossRef]
58. Rumiris, D.; Purwosunu, Y.; Wibowo, N.; Farina, A.; Sekizawa, A. Lower rate of preeclampsia after antioxidant supplementation in pregnant women with low antioxidant status. Hypertens. Pregnancy 2006, 25, 241–253. [CrossRef] [PubMed]
59. Watson, M.; Van Leer, L.; Vanderlelie, J.; Perkins, A. Selenium supplementation protects trophoblast cells from oxidative stress. Placenta 2012, 33, 1012–1019. [CrossRef] [PubMed]
60. Tsuzuki, S.; Morimoto, N.; Hosokawa, S.; Matsushita, T. Associations of maternal and neonatal serum trace element concentrations with neonatal birth weight. PLoS ONE 2013, 8, e75627. [CrossRef] [PubMed]
61. Yao, X.; Ei-Samahy, M.; Fan, L.; Zheng, L.; Jin, Y.; Zhang, G.; Liu, Z.;Wang, F. In vitro influence of selenium on the proliferation of and steroidogenesis in goat luteinized granulosa cells. Theriogenology 2018, 114, 70–80. [CrossRef] [PubMed]
62. Hill, K.E.; Lyons, P.R.; Burk, R.F. Differential regulation of rat liver selenoprotein mRNAs in selenium deficiency. Biochem. Biophys Res. Commun. 1992, 185, 260–263. [CrossRef]
63. Bösl, M.R.; Takaku, K.; Oshima, M.; Nishimura, S.; Taketo, M.M. Early embryonic lethality caused by targeted disruption of the mouse selenocysteine tRNA gene (Trsp). Proc. Natl. Acad. Sci. 1997, 94, 5531–5534. [CrossRef] [PubMed]
64. Lee, A.M.; Huel, G.; Godin, J.; Hellier, G.; Sahuquillo, J.; Moreau, T.; Blot, P. Inter-individual variation of selenium in maternal plasma, cord plasma and placenta. Sci. Total Environ. 1995, 159, 119–127. [CrossRef]
65. Eisenmann, C.; Miller, R. The placental transfer and toxicity of selenite relative to cadmium in the human term perfused placenta. Placenta 1994, 15, 883–895. [CrossRef]
66. Olson, G.E.; Whitin, J.C.; Hill, K.E.; Winfrey, V.P.; Motley, A.K.; Austin, L.M.; Deal, J.; Cohen, H.J.; Burk, R.F. Extracellular glutathione peroxidase (Gpx3) binds specifically to basement membranes of mouse renal cortex tubule cells. Am. J. Physiol. Ren. Physiol. 2009, 298, F1244–F1253. [CrossRef] [PubMed]
67. Burk, R.F.; Hill, K.E. Selenoprotein P—expression, functions, and roles in mammals. Biochim. et Biophys. Acta Gen. Subj. 2009, 1790, 1441–1447. [CrossRef] [PubMed]
68. Burk, R.F.; Hill, K.E. Regulation of selenium metabolism and transport. Annu. Rev. Nutr. 2015, 35, 109–134. [CrossRef] [PubMed]
69. Kurokawa, S.; Hill, K.E.; McDonald, W.H.; Burk, R.F. Long-isoform mouse selenoprotein P (Sepp1) supplies rat myoblast L8 cells with selenium via endocytosis mediated by heparin-binding properties and apolipoprotein E receptor-2 (apoER2). J. Biol. Chem. 2012, 287, 28717–28726. [CrossRef] [PubMed] Molecules 2018, 23, 3053 21 of 24
70. Bou-Resli, M.; Dashti, H.; Mathew, T.; Al-Zaid, N. Pre-and postnatal tissue selenium of the rat in the growing state. Neonatology 2001, 80, 169–172. [CrossRef] [PubMed]
71. Hatzirodos, N.; Hummitzsch, K.; Irving-Rodgers, H.F.; Harland, M.L.; Morris, S.E.; Rodgers, R.J. Transcriptome profiling of granulosa cells from bovine ovarian follicles during atresia. BMC Genomics 2014, 15, 40. [CrossRef] [PubMed]
72. Kasik, J.; Rice, E. Selenoprotein P expression in liver, uterus and placenta during late pregnancy. Placenta 1995, 16, 67–74. [CrossRef]
73. Galton, V.A.; Martinez, E.; Hernandez, A.; St. Germain, E.A.; Bates, J.M.; St. Germain, D.L. The type 2 iodothyronine deiodinase is expressed in the rat uterus and induced during pregnancy. Endocrinology 2001, 142, 2123–2128. [CrossRef] [PubMed]
74. Koopdonk-Kool, J.M.; De Vijlder, J.; Veenboer, G.J.; Ris-Stalpers, C.; Kok, J.H.; Vulsma, T.; Boer, K.; Visser, T.J. Type II and type III deiodinase activity in human placenta as a function of gestational age. J. Clin. Endocrinol. Metab. 1996, 81, 2154–2158. [PubMed]
75. Ejima, K.; Koji, T.; Nanri, H.; Kashimura, M.; Ikeda, M. Expression of thioredoxin and thioredoxin reductase in placentae of pregnant mice exposed to lipopolysaccharide. Placenta 1999, 20, 561–566. [CrossRef] [PubMed]
76. Ejima, K.; Nanri, H.; Toki, N.; Kashimura, M.; Ikeda, M. Localization of thioredoxin reductase and thioredoxin in normal human placenta and their protective effect against oxidative stress. Placenta 1999, 20, 95–101. [CrossRef] [PubMed]
77. Basini, G.; Tamanini, C. Selenium stimulates estradiol production in bovine granulosa cells: possible involvement of nitric oxide. Domes. Anim. Endocrinol. 2000, 18, 1–17. [CrossRef]
78. Grazul-Bilska, A.T.; Caton, J.S.; Arndt, W.; Burchill, K.; Thorson, C.; Borowczyk, E.; Bilski, J.J.; Redmer, D.A.; Reynolds, L.P.; Vonnahme, K.A. Cellular proliferation and vascularization in ovine fetal ovaries: Effects of undernutrition and selenium in maternal diet. Reproduction 2009, 137, 699–707. [CrossRef] [PubMed]
79. Kamada, H.; Ikumo, H. Effect of selenium on cultured bovine luteal cells. Anim. Reprod. Sci. 1997, 46, 203–211. [CrossRef]
80. Tilly, J.L.; Tilly, K. Inhibitors of oxidative stress mimic the ability of follicle-stimulating hormone to suppress apoptosis in cultured rat ovarian follicles. Endocrinology 1995, 136, 242–252. [CrossRef] [PubMed]
81. Grabek, M.; Swies, Z.; Borzecki, A. The influence of selenium on the reproduction of rats. Eur. PMC 1991, 46, 103–105.
82. Özkaya, M.O.; Naz?ro? glu, M.; Barak, C.; Berkkanoglu, M. Effects of multivitamin/mineral supplementation on trace element levels in serum and follicular fluid of women undergoing in vitro fertilization (IVF). Biol. Trace Elem. Res. 2011, 139, 1–9. [CrossRef] [PubMed]
83. Ruder, E.H.; Hartman, T.J.; Goldman, M.B. Impact of oxidative stress on female fertility. Curr. Opin. Obstet. Gynecol. 2009, 21, 219–222. [CrossRef] [PubMed]
84. Mehdi, Y.; Hornick, J.-L.; Istasse, L.; Dufrasne, I. Selenium in the environment, metabolism and involvement in body functions. Molecules 2013, 18, 3292–3311. [CrossRef] [PubMed]
85. Edassery, S.L.; Shatavi, S.V.; Kunkel, J.P.; Hauer, C.; Brucker, C.; Penumatsa, K.; Yu, Y.; Dias, J.A.; Luborsky, J.L. Autoantigens in ovarian autoimmunity associated with unexplained infertility and premature ovarian failure. Fertil. Steril. 2010, 94, 2636–2641. [CrossRef] [PubMed]
86. Singh, A.K.; Chattopadhyay, R.; Chakravarty, B.; Chaudhury, K. Markers of oxidative stress in follicular fluid of women with endometriosis and tubal infertility undergoing IVF. Reprod. Toxicol. 2013, 42, 116–124. [CrossRef] [PubMed]
87. Coskun, A.; Arikan, T.; Kilinc, M.; Arikan, D.C.; Ekerbiçer, H.Ç. Plasma selenium levels in Turkish women with polycystic ovary syndrome. Eur. J. Obstet. Gynecol. Reprod. Biol. 2013, 168, 183–186. [CrossRef] [PubMed]
88. Luddi, A.; Capaldo, A.; Focarelli, R.; Gori, M.; Morgante, G.; Piomboni, P.; De Leo, V. Antioxidants reduce oxidative stress in follicular fluid of aged women undergoing IVF. Reprod. Biol. Endocrinol. 2016, 14, 57. [CrossRef] [PubMed]
89. Jiménez Tuñón, J.M.; Trilles, P.P.; Molina, M.G.; Duvison, M.H.; Pastor, B.M.; Martín, P.S.; Martín, F.S.; Sánchez-Borrego, R. A Double-Blind, Randomized Prospective Study to Evaluate the Efficacy of Previous TherapyWith Melatonin, Myo-inositol, Folic Acid, and Selenium in Improving the Results of an Assisted Reproductive Treatment. Clin. Med. Insight. Ther. 2017, 9, 1179559X17742902. [CrossRef] Molecules 2018, 23, 3053 22 of 24
90. Wilson, R.L.; Bianco-Miotto, T.; Leemaqz, S.Y.; Grzeskowiak, L.E.; Dekker, G.A.; Roberts, C.T. Early pregnancy maternal trace mineral status and the association with adverse pregnancy outcome in a cohort of Australian women. J. Trace Elem. Med. Biol. 2018, 46, 103–109. [CrossRef] [PubMed]
91. Parman, T.; Wiley, M.J.; Wells, P.G. Free radical-mediated oxidative DNA damage in the mechanism of thalidomide teratogenicity. Nat. Med. 1999, 5, 582–585. [CrossRef] [PubMed]
92. Winn, L.M.; Wells, P.G. Maternal administration of superoxide dismutase and catalase in phenytoin teratogenicity1. Free Radical Biol. Med. 1999, 26, 266–274. [CrossRef]
93. Thompson, J. In vitro culture and embryo metabolism of cattle and sheep embryos—a decade of achievement. Anim. Reprod. Sci. 2000, 60, 263–275. [CrossRef]
94. Uhm, S.J.; Gupta, M.K.; Yang, J.H.; Lee, S.H.; Lee, H.T. Selenium improves the developmental ability and reduces the apoptosis in porcine parthenotes. Mol. Reprod. Dev. 2007, 74, 1386–1394. [CrossRef] [PubMed]
95. McKeehan, W.L.; Hamilton, W.G.; Ham, R.G. Selenium is an essential trace nutrient for growth of WI-38 diploid human fibroblasts. Proc. Natl. Acad. Sci. 1976, 73, 2023–2027. [CrossRef] [PubMed]
96. Hewlett, G. Strategies for optimising serum-free media. Cytotechnology 1991, 5, 3–14. [CrossRef] [PubMed]
97. Ufer, C.; Wang, C.C. The roles of glutathione peroxidases during embryo development. Front. Mol. Neurosci. 2011, 4, 12. [CrossRef] [PubMed]
98. Yoshida, M.; Ishigaki, K.; Nagai, T.; Chikyu, M.; Pursel, V.G. Glutathione concentration during maturation and after fertilization in pig oocytes: relevance to the ability of oocytes to form male pronucleus. Biol. Reprod. 1993, 49, 89–94. [CrossRef] [PubMed]
99. Pierce, G.B.; Parchment, R.E.; Lewellyn, A.L. Hydrogen peroxide as a mediator of programmed cell death in the blastocyst. Differentiation 1991, 46, 181–186. [CrossRef] [PubMed]
100. Parchment, R. The implications of a unified theory of programmed cell death, polyamines, oxyradicals and histogenesis in the embryo. Int. J. Dev. Biol. 2003, 37, 75–83.
101. Abedelahi, A.; Salehnia, M.; Allameh, A.; Davoodi, D. Sodium selenite improves the in vitro follicular development by reducing the reactive oxygen species level and increasing the total antioxidant capacity and glutathione peroxide activity. Hum. Reprod. 2010, 25, 977–985. [CrossRef] [PubMed]
102. Abedelahi, A.; Salehnia, M.; Allameh, A. The effects of different concentrations of sodium selenite on the in vitro maturation of preantral follicles in serum-free and serum supplemented media. J. Assist. Reprod. Genet. 2008, 25, 483–488. [CrossRef] [PubMed]
103. Shi, L.; Ren, Y.; Zhang, C.; Yue, W.; Lei, F. Effects of organic selenium (Se-enriched yeast) supplementation in gestation diet on antioxidant status, hormone profile and haemato-biochemical parameters in Taihang Black Goats. Anim. Feed Sci. Technol. 2018, 238, 57–65. [CrossRef]
104. Dalto, B.; Tsoi, S.; Audet, I.; Dyck, M.K.; Foxcroft, G.; Matte, J.J. Gene expression of porcine blastocysts from gilts fed organic or inorganic selenium and pyridoxine. Reproduction 2015, 149, 31–42. [CrossRef] [PubMed]
105. Dalto, D.B.; Audet, I.; Lapointe, J.; Matte, J.J. The importance of pyridoxine for the impact of the dietary selenium sources on redox balance, embryo development, and reproductive performance in gilts. J. Trace Elem. Med. Biol. 2016, 34, 79–89. [CrossRef] [PubMed]
106. Dalto, D.B.; Roy, M.; Audet, I.; Palin, M.-F.; Guay, F.; Lapointe, J.; Matte, J.J. Interaction between vitamin B6 and source of selenium on the response of the selenium-dependent glutathione peroxidase system to oxidative stress induced by oestrus in pubertal pig. J. Trace Elem. Med. Biol. 2015, 32, 21–29. [CrossRef] [PubMed]
107. Fortier, M.E.; Audet, I.; Giguere, A.; Laforest, J.P.; Bilodeau, J.F.; Quesnel, H.; Matte, J.J. Effect of dietary organic and inorganic selenium on antioxidant status, embryo development, and reproductive performance in hyperovulatory first-parity gilts. J. Anim. Sci. 2012, 90, 231–240. [CrossRef] [PubMed]
108. Chen, J.; Han, J.; Guan, W.; Chen, F.; Wang, C.; Zhang, Y.; Lv, Y.; Lin, G. Selenium and vitamin E in sow diets: I. Effect on antioxidant status and reproductive performance in multiparous sows. Anim. Feed Sci. Technol. 2016, 221, 111–123. [CrossRef]
109. Nogales, F.; Ojeda, M.L.; Fenutría, M.; Murillo, M.L.; Carreras, O. Role of selenium and glutathione peroxidase on development, growth, and oxidative balance in rat offspring. Reproduction 2013, 146, 659–667. [CrossRef] [PubMed]
110. Khera, A.; Dong, L.-F.; Holland, O.; Vanderlelie, J.; Pasdar, E.A.; Neuzil, J.; Perkins, A.V. Selenium supplementation induces mitochondrial biogenesis in trophoblasts. Placenta 2015, 36, 863–869. [CrossRef] [PubMed] Molecules 2018, 23, 3053 23 of 24
111. Khera, A.; Vanderlelie, J.J.; Holland, O.; Perkins, A.V. Overexpression of endogenous anti-oxidants with selenium supplementation protects trophoblast cells from reactive oxygen species-induced apoptosis in a Bcl-2-dependent manner. Biol. Trace Elem. Res. 2017, 177, 394–403. [CrossRef] [PubMed]
112. Perkins, A.; Khera, A.; Holland, O.; Vanderlelie, J. Trophoblast mitochondrial biogenesis and functionality is increased with selenium supplementation. Placenta 2016, 45, 118. [CrossRef]
113. Mendelev, N.; Mehta, S.L.; Idris, H.; Kumari, S.; Li, P.A. Selenite stimulates mitochondrial biogenesis signaling and enhances mitochondrial functional performance in murine hippocampal neuronal cells. PLoS ONE 2012, 7, e47910. [CrossRef] [PubMed]
114. Na, J.Y.; Seok, J.; Park, S.; Kim, J.S.; Kim, G.J. Effects of selenium on the survival and invasion of trophoblasts. Clin. Exp. Reprod. Med. 2018, 45, 10–16. [CrossRef] [PubMed]
115. Fialova, L.; Malbohan, I.; Kalousova, M.; Soukupova, J.; Krofta, L.; Štipek, S.; Zima, T. Oxidative stress and inflammation in pregnancy. Scand. J. Clin. Lab. INVEST. 2006, 66, 121–128. [CrossRef] [PubMed]
116. Orhan, H.; Önderoglu, L.; Yücel, A.; Sahin, G. Circulating biomarkers of oxidative stress in complicated pregnancies. Arch. Gynecol. Obst. 2003, 267, 189–195.
117. Karowicz-Bilinska, A.; K?edziora-Kornatowska, K.; Bartosz, G. Indices of oxidative stress in pregnancy with fetal growth restriction. Free Radical Res. 2007, 41, 870–873. [CrossRef] [PubMed]
118. Hracsko, Z.; Orvos, H.; Novak, Z.; Pal, A.; Varga, I.S. Evaluation of oxidative stress markers in neonates with intra-uterine growth retardation. Redox Rep. 2008, 13, 11–16. [CrossRef] [PubMed]
119. Mariath, A.B.; Bergamaschi, D.P.; Rondó, P.H.; Ana, C.A.T.; de Fragas Hinnig, P.; Abbade, J.F.; Diniz, S.G. The possible role of selenium status in adverse pregnancy outcomes. Br. J. Nutr. 2011, 105, 1418–1428. [CrossRef] [PubMed]
120. Richard, K.; Holland, O.; Landers, K.; Vanderlelie, J.J.; Hofstee, P.; Cuffe, J.S.; Perkins, A.V. Effects of maternal micronutrient supplementation on placental function. Placenta 2017, 54, 38–44. [CrossRef] [PubMed]
121. Mamon, M.A.C.; Ramos, G.B. Maternal selenium-supplementation at various stages of periconception period: influence on murine blastocyst morphology and implantation status. J. Anim. Sci. Technol. 2017, 59, 7. [CrossRef] [PubMed]
122. Cetin, I.; Berti, C.; Calabrese, S. Role of micronutrients in the periconceptional period. Hum. Reprod. Update 2009, 16, 80–95. [CrossRef] [PubMed]
123. Berti, C.; Biesalski, H.; Gärtner, R.; Lapillonne, A.; Pietrzik, K.; Poston, L.; Redman, C.; Koletzko, B.; Cetin, I. Micronutrients in pregnancy: current knowledge and unresolved questions. Clin. Nutr. 2011, 30, 689–701. [CrossRef] [PubMed]
124. Ambroziak, U.; Hybsier, S.; Shahnazaryan, U.; Krasnod?ebska-Kilja ´ nska, M.; Rijntjes, E.; Bartoszewicz, Z.; Bednarczuk, T.; Schomburg, L. Severe selenium deficits in pregnant women irrespective of autoimmune thyroid disease in an area with marginal selenium intake. J. Trace Elem. Med. Biol. 2017, 44, 186–191. [CrossRef] [PubMed]
125. Polanska, K.; Krol, A.; Sobala,W.; Gromadzinska, J.; Brodzka, R.; Calamandrei, G.; Chiarotti, F.;Wasowicz,W.; Hanke, W. Selenium status during pregnancy and child psychomotor development—Polish Mother and Child Cohort study. Pediatr. Res. 2016, 79, 863. [CrossRef] [PubMed]
126. Butler, J.A.; Whanger, P.D.; Tripp, M.J. Blood selenium and glutathione peroxidase activity in pregnant women: comparative assays in primates and other animals. Am. J. Clin. Nutr. 19821982, 36, 15–23. [CrossRef] [PubMed]
127. Zachara, B.; Wardak, C.; Didkowski, W.; Maciag, A.; Marchaluk, E. Changes in blood selenium and glutathione concentrations and glutathione peroxidase activity in human pregnancy. Gynecol. Obst. Invest. 1993, 35, 12–17. [CrossRef] [PubMed]
128. Jarvis, S.; Glinianaia, S.V.; Torrioli, M.-G.; Platt, M.-J.; Miceli, M.; Jouk, P.-S.; Johnson, A.; Hutton, J.; Hemming, K.; Hagberg, G. Cerebral palsy and intrauterine growth in single births: European collaborative study. The Lancet 2003, 362, 1106–1111. [CrossRef]
129. Dikbas, L.; Yapca, O.E.; Dikbas, N.; Gundogdu, C. Paraoxonase-2 and paraoxonase-3: comparison of mRNA expressions in the placentae of unexplained intrauterine growth restricted and noncomplicated pregnancies. J. Mat.-Fet. Neonatal Med. 2017, 30, 1200–1206. [CrossRef] [PubMed]
130. Takagi, Y.; Nikaido, T.; Toki, T.; Kita, N.; Kanai, M.; Ashida, T.; Ohira, S.; Konishi, I. Levels of oxidative stress and redox-related molecules in the placenta in preeclampsia and fetal growth restriction. Virchows Arch. 2004, 444, 49–55. [CrossRef] [PubMed] Molecules 2018, 23, 3053 24 of 24
131. Mesdaghinia, E.; Rahavi, A.; Bahmani, F.; Sharifi, N.; Asemi, Z. Clinical and Metabolic Response to Selenium Supplementation in PregnantWomen at Risk for Intrauterine Growth Restriction: Randomized, Double-Blind, Placebo-Controlled Trial. Biol. Trace Elem. Res. 2017, 178, 14–21. [CrossRef] [PubMed]
132. Barrington, J.; Lindsay, P.; James, D.; Smith, S.; Roberts, A. Selenium deficiency and miscarriage: A possible link? Int. J. Obstet. Gynaecol. 1996, 103, 130–132. [CrossRef]
133. Koçak, ˙I.; Aksoy, E.; Üstün, C. Recurrent spontaneous abortion and selenium deficiency. Int. J. Obstet. Gynaecol. 1999, 65, 79–80. [CrossRef]
134. Mistry, H.D.; Kurlak, L.O.; Young, S.D.; Briley, A.L.; Broughton Pipkin, F.; Baker, P.N.; Poston, L. Maternal selenium, copper and zinc concentrations in pregnancy associated with small-for-gestational-age infants. Mat. Child Nutr. 2014, 10, 327–334. [CrossRef] [PubMed]
135. Negro, R.; Greco, G.; Mangieri, T.; Pezzarossa, A.; Dazzi, D.; Hassan, H. The influence of selenium supplementation on postpartum thyroid status in pregnant women with thyroid peroxidase autoantibodies. J. Clin. End. Metab. 2007, 92, 1263–1268. [CrossRef] [PubMed]
136. Rayman, M.P. Multiple nutritional factors and thyroid disease, with particular reference to autoimmune thyroid disease. Proc. Nutr. Soci. 2018, 1–11. [CrossRef] [PubMed]
137. Brigelius-Flohé, R.; Arnér, E.S. Selenium and selenoproteins in (redox) signaling, diseases, and animal models-200 year anniversary issue. Eur. PMC 2018, 127, 1–2. [CrossRef] [PubMed]
138. Wu,W.;Wang, Q.; Yin, F.; Yang, Z.; Zhang,W.; Gabra, H.; Li, L. Identification of proteomic and metabolic signatures associated with chemoresistance of human epithelial ovarian cancer. Int. J. Clin. Oncol. 2016, 49, 1651–1665. [CrossRef] [PubMed]
139. Agnani, D.; Camacho-Vanegas, O.; Camacho, C.; Lele, S.; Odunsi, K.; Cohen, S.; Dottino, P.; Martignetti, J.A. Decreased levels of serum glutathione peroxidase 3 are associated with papillary serous ovarian cancer and disease progression. J. Ovarian Res. 2011, 4, 18. [CrossRef] [PubMed]
140. Piekutowski, K.; Makarewicz, R.; Zachara, B. The antioxidative role of selenium in pathogenesis of cancer of the female reproductive system. Neoplasma 2007, 54, 374–378. [PubMed]
141. Falck, E.; Karlsson, S.; Carlsson, J.; Helenius, G.; Karlsson, M.; Klinga-Levan, K. Loss of glutathione peroxidase 3 expression is correlated with epigenetic mechanisms in endometrial adenocarcinoma. Cancer Cell Int. 2010, 10, 46. [CrossRef] [PubMed]
142. Saga, Y.; Ohwada, M.; Suzuki, M.; Konno, R.; Kigawa, J.; Ueno, S.; Mano, H. Glutathione peroxidase 3 is a candidate mechanism of anticancer drug resistance of ovarian clear cell adenocarcinoma. Oncol. Rep. 2008, 20, 1299–1303. [CrossRef] [PubMed]
143. Lee, H.J.; Do, J.H.; Bae, S.; Yang, S.; Zhang, X.; Lee, A.; Choi, Y.J.; Park, D.C.; Ahn,W.S. Immunohistochemical evidence for the over-expression of Glutathione peroxidase 3 in clear cell type ovarian adenocarcinoma. Med. Oncol. 2011, 28, 010–9659. [CrossRef] [PubMed]
144. Hough, C.D.; Cho, K.R.; Zonderman, A.B.; Schwartz, D.R.; Morin, P.J. Coordinately up-regulated genes in ovarian cancer. Cancer Res. 2001, 61, 3869–3876. [PubMed]
145. Rayman, M.P.; Winther, K.H.; Pastor-Barriuso, R.; Cold, F.; Thvilum, M.; Stranges, S.; Guallar, E.; Cold, S. Effect of long-term selenium supplementation on mortality: results from a multiple-dose, randomized controlled trial. Free Radical Biol. Med. 2018. [CrossRef] [PubMed]
关键词:女性生育;繁殖;GPX1;不孕;卵巢功能;氧化应激;子痫前期;硒;硒蛋白
1. 引言
硒(Se)是一种在分子和组织水平上对动物和人体健康具有多种重要功能的必需微量元素,与多种病理生理学相关[1]。硒的生物功能主要通过硒蛋白(由25种人类基因和24种小鼠基因编码的含硒蛋白)形式来实现,报道显示,真核生物、古细菌、真细菌这三个生命领域都存在硒蛋白,此外,在病毒中也发现了硒蛋白的存在[2-4]。作为硒蛋白的组成成分,硒具有结构功能和酶相关功能。在后者中硒的催化和抗氧化活性被广泛认知[1]。
所有发现的哺乳动物的硒蛋白中,只有少数硒蛋白被发现具有功能活性[4]。虽然这些硒蛋白作用的分子通路和所具有重要生物功能都各有不同,但它们至少都含有一种硒代半胱氨酸(Sec,一种含硒氨基酸,它是第21种自然形成的氨基酸,与其它翻译后修饰的氨基酸不同,硒代氨基酸能通过生物合成嵌入到蛋白质中[3,5]),并且其中大部分都能发挥氧化还原酶的作用[4,6]。包括DNA的脱氧核糖核苷合成、清除有害过氧化物、被氧化的蛋白和细胞膜的还原、调控氧化还原信号、甲状腺激素代谢、蛋白质折叠和硒的运输和贮存在内的多种重要细胞生理活动均被发现需依赖于硒蛋白进行[4]。在此,我们选择了一些与繁育、生殖和发育相关的哺乳动物硒蛋白,并简述总结于表1中。
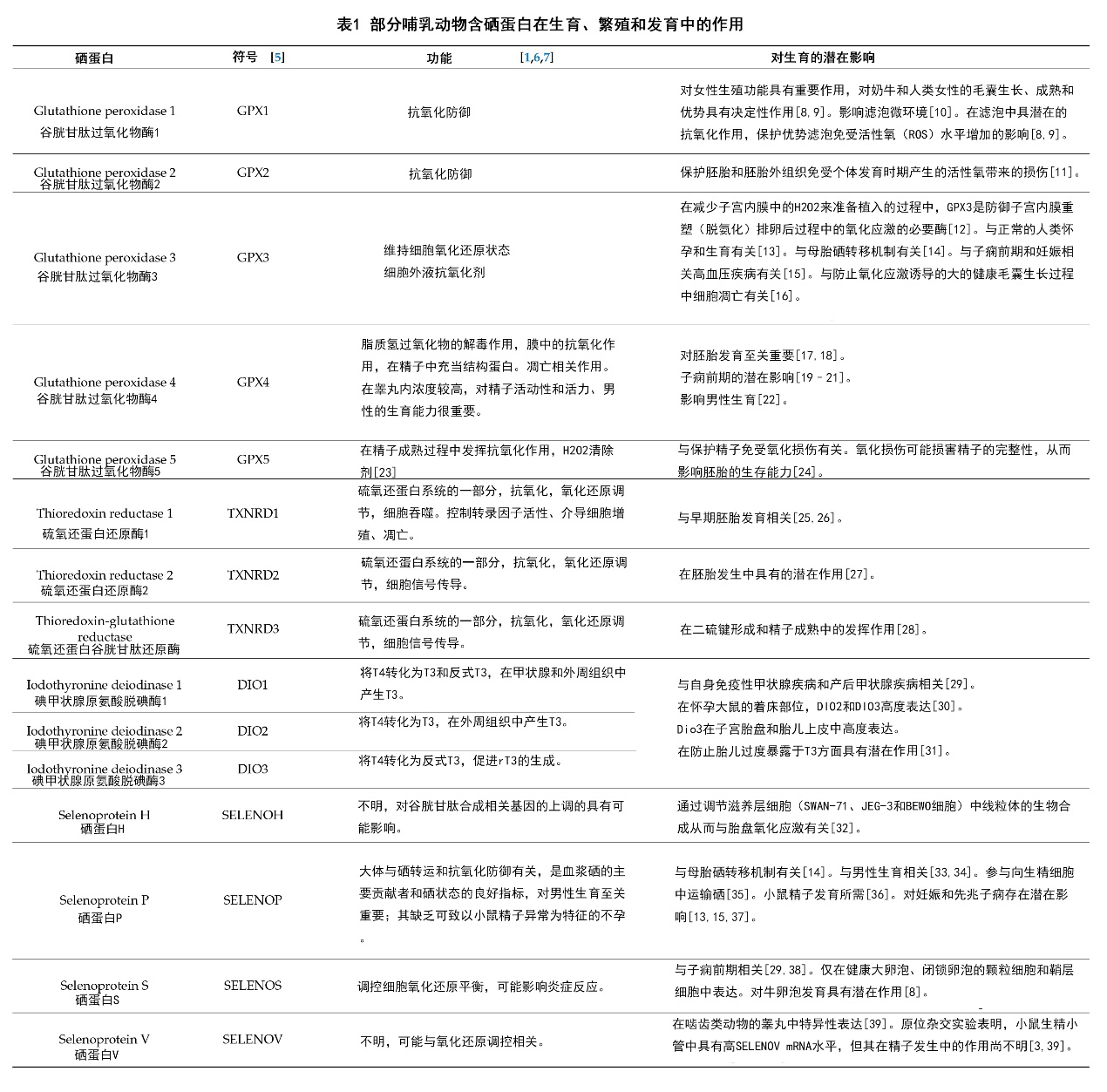
硒通常以有机硒或无机硒的形式被摄取。硒形态对其在健康和组织生长方面的潜在益处和或毒性效应有重要影响[7,40,41]。因此,对植源和动物源食物中的硒形态的考量是十分重要的,此外,我们还需要对硒被植物吸收,以及在动物体内代谢的相关生物机制进行阐明[42]。近期研究发现,膳食硒的总摄入量和硒形态均对健康效应有着重要影响,不过,硒形态对健康效应的影响可能会相对更大[42]。不同哺乳动物的硒推荐摄入量可见表2。
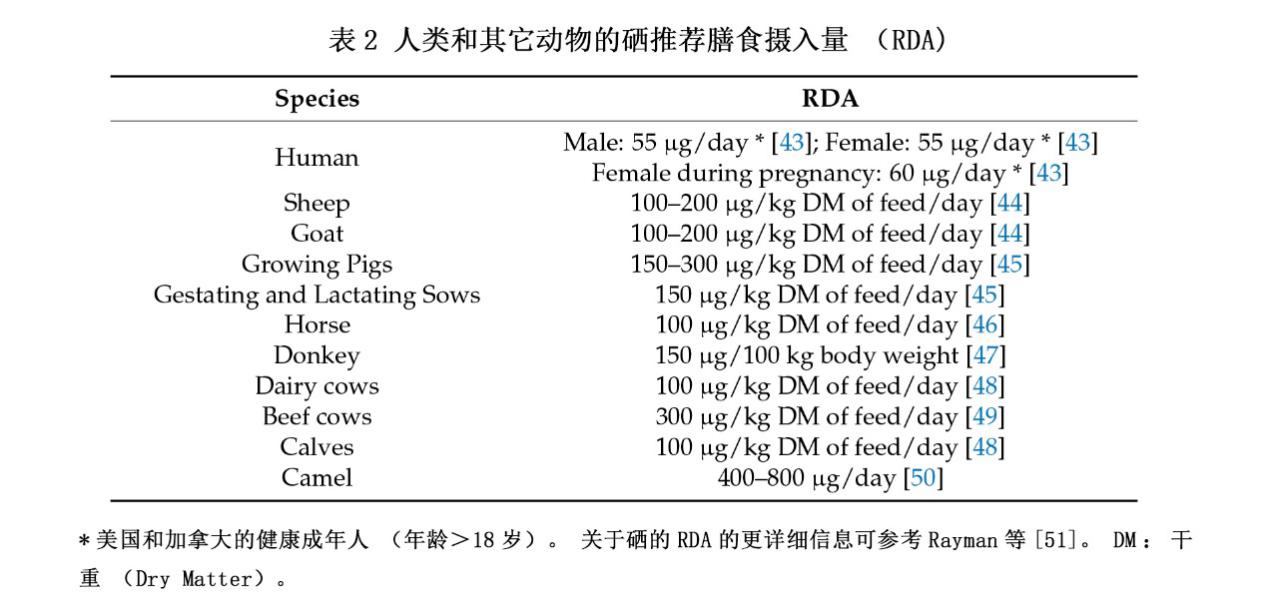
一些哺乳动物研究验证了硒水平与男性[52,53]、女性[53,54]生殖功能之间的关系。现有证据表明,硒在多种不良妊娠健康情况中具有重要意义,如子痫前期,自身免疫性甲状腺疾病、流产和早产[29]。此外,缺硒或许对生育率下降、胎盘滞留和生殖疾病的发生(如子宫炎)具有重要影响[55]。硒增加带来的生育率的增加或可归因于妊娠30天内的胚胎死亡率的下降[55]。大多数的女性研究主要集中在硒在妊娠中的作用[56-60],但最近也有关于硒在卵母细胞的发展和卵巢生理方面的潜能的相关研究被报道[8,53,61]。
2.硒和硒蛋白的运输
研究显示,缺硒会导致硒蛋白浓度的下降[62]。Bosl及其同事发现,纯合子Trsp(-/-)胚胎合成硒蛋白的能力低下促进了其胚胎死亡[63]。硒蛋白合成由tRNASec介导,然而tRNASec自身的数量并不太可能限制硒蛋白合成,反而硒自身的可用性可能发挥了重要作用[63]。因此,孕妇为胚胎或胎儿提供硒对硒蛋白的合成是必需的[14]。硒经胎盘进行的运输具有双向性,或会影响硒在孕妇、胎儿和新生儿组织中的净滞留[7,64]。硒是否容易穿过女性胎盘组织或渗透包含在胎盘组织中目前仍不太清楚[7,65]。小鼠血浆中的两种细胞外硒蛋白(硒蛋白P和谷胱甘肽过氧化物酶3(Gpx3))含有97%的硒[14,66]。硒蛋白P主要在肝脏中合成,再将硒运往其它大部分组织[14,67],然而关于Gpx3在硒运输方面的作用的信息却相对缺乏[14]。硒蛋白P的运输由载脂蛋白E受体2所介导,后者可作为体循环中的硒发生细胞內吞作用的媒介[14]。与其它组织和器官中的apoER2类似,胎盘中的apoER2似乎也能摄入硒蛋白P[14]。最近有报道表明硒蛋白P和Gpx3都可能参与了硒从孕妇到发育中的胚胎组织器官中的转运[14]。这些发现为硒的转运机制(卵黄囊与胎盘机制)提供了思路[14]。
小鼠实验发现,胚胎卵黄囊介导的母-胎硒转运发生在妊娠早期,晚期则通过胎盘进行[14,68]。胚胎卵黄囊能吸收子宫中含有硒蛋白P和Gpx3的液体进而实现硒运输,而胎盘则通过apoER2介导的细胞內吞作用吸收母体血液中的硒蛋白P[14]。这两种吸收机制都依赖于硒蛋白并需在特殊机体环境下进行(如缺硒)[14]。然而,也有研究表明硒的这种运输也可独立于硒蛋白P和Gpx3(血浆硒蛋白)进行[14]。硒蛋白P和Gpx3基因敲除小鼠能够在高硒饮食中存活,表明硒到组织还有其它运输途径[14,66,68]。由此可推断或许存在两种特定水平下的硒转运机制:低级机制和高级机制[14]。低级机制(可能涉及硒多糖或其它小分子硒化物)似乎并不直接依赖于硒蛋白[14],而高度依赖于妊娠母体的硒水平,这种机制降低了缺硒条件下的转运效率和在硒充足条件下的有效性 [14,68]。另一方面,高级机制(硒蛋白P-apoER2机制[69])即使在膳食硒不足的情况下也能够根据需求将硒运输到靶向组织中[14]。Burk等人发现胚胎卵黄囊介导的硒运输就具有高等运输机制的特点[14]。虽然这种运输不由硒蛋白受体介导,但它明显依赖于细胞对硒蛋白摄入,并在硒缺乏条件下也依然有效[14]。因此,无论是胚胎卵黄囊还是胎盘都能通过高等机制来实现母-胎硒运输(硒蛋白依赖),从而为缺硒孕妇的胎儿提供保护[14,68]。
3.硒蛋白在女性生殖器官中的表达
无机硒和有机硒均与硒蛋白P的表达调控相关[7]。研究发现,妊娠期大鼠的胎盘中的硒含量和硒蛋白的表达水平会同时上升[7]。许多硒蛋白如硒蛋白P、碘甲状腺原氨酸脱碘酶3(Dio3)和硫氧还蛋白还原酶(Txnrd)均会在子宫内表达[7,70]。同时,有证据发现GPX1、GPX2、GPX3、GPX4、SELENOS(也被称为VIMP)、SELENOT、SELENOF、SELENOH、SEPHS2、DIO3、DIO1和SELENOM等其它硒蛋白在牛卵巢颗粒细胞中也会表达[8]。小的、闭塞的牛卵泡中观察到了健康牛卵泡所没有的硒蛋白P表达上调和GPX1、GPX3表达下调[71]。在妊娠期小鼠中还观察到了胎盘和子宫中硒蛋白P的表达改变[7,72]。足月小鼠生产前四天其硒蛋白水平出现了不寻常地增长,并达到了峰值[72]。此外,硒蛋白在小鼠胎儿肝脏中也被发现具有早期表达[72]。
甲状腺激素对哺乳动物的生育能力尤为重要,贯穿了胚胎发育始终[73]。在人类和啮齿动物中,缺乏甲状腺激素与生育能力降低、发情周期失调、子宫结构与着床受损,以及其它哺乳动物妊娠问题相关[73]。Galton及其同事发现,妊娠小鼠的子宫中的Dio3 mRNA具有显著更高水平的表达[30]。Dio3的表达具有时间和区域特异性,例如,妊娠第九天发现子宫中层和抗中层蜕膜中存在Dio3的高度表达[7,30]。此外,着床位置也可观察到Dio3 mRNA及其相关活动[30]。Dio3在着床位置上的这种高水平表达可能是出于对暴露于母体甲状腺激素中的胎儿发育的一种保护[7,30]。此外,在人类胎盘细胞中,Dio3活性随胎龄增加而增加[7,74]。有力证据表明,妊娠期的胎盘组织在调节胎儿在母体甲状腺激素中的暴露水平方面发挥着重要作用[30]。此外,在合胞体滋养层和细胞滋养层、胎盘血管内皮层和脐带羊膜、子宫蜕膜、胎儿上皮和非妊娠子宫内膜中也发现了较高的Dio3表达[31]。这些研究结果表明,甲状腺激素水平的区域性调控对女性生育的全部阶段都具有重要作用[31]。其它一些因素也被认为对胎盘和子宫具有影响[7]。硫氧还蛋白还原酶(TPX)和TXNRD在人类和啮齿动物胎盘中也具有区域特异性表达[7,75,76]。组织化学观察表明,这两种蛋白酶都集中在子宫内膜和干细胞间质细胞的胚胎滋养层和上皮组织中[7,75,76],有研究发现,这两种蛋白能在炎症环境下保护胎盘组织。
4.硒在卵泡发育和卵巢功能中的作用
卵巢颗粒细胞的生长是卵泡发育过程(如卵泡形成)的一个重要特征。初级小滤泡的增殖(伴随着更少的卵巢颗粒细胞)发育为排卵前期的成熟卵泡(伴随着多层细胞)是卵泡生成过程中的特征性事件[77]。动物实验发现,硒也许能调控卵巢颗粒细胞的生长,成人卵巢体外实验发现,硒或许还能调控17β-雌二醇的生物合成[77]。近期研究也证实了在健康卵泡中硒和硒蛋白水平的上升,这也许是为了在卵泡后期和增殖阶段提供重要的抗氧化功能[8]。然而,人们对硒在卵巢功能和发育中的这种调节作用现在仍知之甚少[78]。Grazul-Bilska及其同事研究发现,相较于硒适当组(6μg/kg bw),孕期膳食中的高硒水平(80μg/kg bw)抑制了初级、次生、窦卵泡、基质和羊水中胎儿血管系统的生长。伴随着高硒饮食,原始卵泡增殖数和原始卵泡标记指数(增殖细胞百分比)出现下降[78]。
4.1 体外和动物模型试验证据
亚硒酸钠(无机硒)不仅能促进卵母细胞生长,还能提高膜细胞和颗粒细胞的增殖率。为了验证这一假设,Basini和Tamanini进行了一项体外实验,结果表明补充亚硒酸钠(5ng/ml)促进了牛颗粒细胞的增殖,并对E2合成起到了一定刺激作用。这些作用可以至少部分通过抑制一氧化氮的生成来实现[77]。此外,这些作用还可能受到了颗粒细胞中的某些未知的硒依赖性抗氧化酶的影响[77]。在另一项体外实验中,Kamada和Ikumo发现在培养基中添加5和200ppb的硒能促进牛黄体细胞的增殖,并具有抑制ROS危害的潜力[79]。GPX还被发现能刺激FSH的活性来抑制培养的大鼠卵巢中卵泡的凋亡[80]。另外,缺硒被证明能促进大鼠的卵巢退化和卵泡闭锁[81]。最近,Yao及其同事进行了一项体外研究来阐明硒在山羊黄体颗粒细胞增殖和甾体激素生物合成中作用的潜在机制[61]。增殖细胞核抗原(PCNA)、Akt、磷脂酰肌醇3激酶(PI3K)等生物标记物表主要在卵巢卵母细胞和颗粒细胞中表达[61]。黄体颗粒细胞增殖能被5ng/ml硒显著刺激[61]。这种增殖或许是通过上调PCNA、周期依赖激酶1(CDK1)、磷酸腺苷活化蛋白激酶(p-AMPK,Thr172)和磷酸化Akt(p-Akt,Ser473)等生物标记物的表达和下调p21的表达来实现的[61]。同样,用硒处理还促进了雌二醇分泌,并显著上调了抗氧化酶(GPX和SOD2)和甾类生成相关基因(3β-HSD和StAR)的表达[61]。此外,最近还有研究聚焦于硒在牛卵巢组织中的生物积累[8]。Ceko及其同事通过射线荧光成像技术成功在大的、健康的卵泡中鉴定出了硒,同时,与黄体相比,在牛卵泡的细胞膜上鉴定出了高出黄体10倍左右的硒含量[8]。这些发现均表明,抗氧化型的硒蛋白主要在卵泡中发挥潜在作用,例如,在升高的ROS及其相关氧化损伤中保护卵泡。
4.2 人类研究证据
女性生殖与硒水平、硒依赖性GPX催化活性之间的关系在极少数的几项研究中得到了验证,这些研究发现,低硒与低滤泡液水平与更高的不孕症发生有关[82]。1995年,Paszkowski及其同事首次在人类滤泡液中发现了硒,其中的酶活性表现出硒依赖性[10]。研究者们还评估了来自112位患者阴道卵母细胞的135个滤泡液样本,发现相较于那些知晓病因的不孕症患者(如输卵管不孕或与男性因素相关的不孕),具有特发性不孕症的患者的滤泡硒水平显著降低[10]。基于该发现,研究者们推测,滤泡微环境中的含硒酶GPX的抗氧化活性或许在配子发生和受精过程中发挥了重要作用[10]。最近,Ceko的研究团队提出GPX1在测定卵泡生长、成熟和优势中具有重要作用[8]。基于此,源自卵丘卵母细胞复合体(COCs)的人类卵丘细胞(CCs)被用于体外受精(IVF)和卵母细胞胞浆内单精子注射[8]。这些在胚胎移植前从COCs中恢复的CCs在妊娠期中的GPX1表达明显高于那些妊娠失败者[8]。许多研究者们还发现在膳食硒缺乏的情况下会出现GPX1表达下降,这表明部分卵巢疾病也许能通过补硒进行改善[8]。这些发现将会成为饮食硒摄入、GPX1的体内表达、排卵相关疾病等相关流行病学研究的基础[8,9]。
5. 硒在卵巢病理及辅助生殖技术相关的氧化应激中的意义
氧化应激、女性生育能力降低和缺硒之间的相关性是另一个需要我们调查和研究的领域[83]。一个有益的事实是,子宫内膜异位症(如多囊性子宫内膜异位症)中ROS的过度生成可能导致硒的过度消耗(因硒的抗氧化作用)。低硒水平则会导致伴随着ROS增加的氧化还原水平失衡,后者被认为是多囊卵巢综合症的诱发因素。无论如何,机体的低硒水平会导致对自由基诱导损伤的防御下降,这或许可归因于硒参与硒蛋白的形成并与其抗氧化能力密切相关[84]。
5.1 人类研究
对患有不孕症或原发性卵巢功能不全(POI)的卵巢自身免疫性患者的鉴定也许可借鉴化疗、环境、遗传和辐射相关因素患者并寻找替代治疗方案[85]。硒结合蛋白1(SBP1)对卵巢自身免疫相关的不孕症和POI具有特殊意义[85]。Edassery及其同事在特发性不孕症的妇女中发现了与血清自身抗体相关的抗原分子。在患有特发性不孕症和卵巢功能早衰的女性中发现了明显更高水平的SBP1[85]。印度的Singh等人研究了经历过体外受精(IVF)的子宫内膜异位症和输卵管不孕症的女性的滤泡液中的氧化应激标记物,结果发现与对照相比,子宫内膜异位症患者的滤泡液中的ROS和丙二醛生成显著增加[86]。此外,相较于长期输卵管不孕不育的女性,子宫内膜异位症合并不孕的女性滤泡液中的硒水平显著降低[86]。2013年土耳其的一项研究表明,36位患有多囊卵巢综合征的女性的血浆硒水平显著低于对照组(n=33,BMI健康女性)[87]。这些多囊卵巢综合征女性患者的硒水平降低可能与高雄激素血症有关[87]。
此外,适量补充包括硒在内的微量元素被发现也许能显著影响滤泡微环境中的氧化还原平衡,从而影响IVF结果[88]。最近两项关于ART的临床试验(Luddi等[88]和Jimenez Tunon等[89])发现,补硒(以微量元素形式)也许能改善IVF结局(表3)。这些研究均因其样本量过少而存在局限性,另一局限性在于这两项实验均是将硒与其它微量元素合用进行补充,因而不能完全排除其它微量元素的影响。这些发现或许能在一定程度上为临床医生应对经IVF治疗的女性时提供一些参考[88]。然而,我们仍需对孕妇在怀孕期间的营养进行的机械和流行病学研究,特别是对多种微量营养素及其相互作用的研究,来充分阐明这些因素在成功生育和怀孕中的重要性[90]。
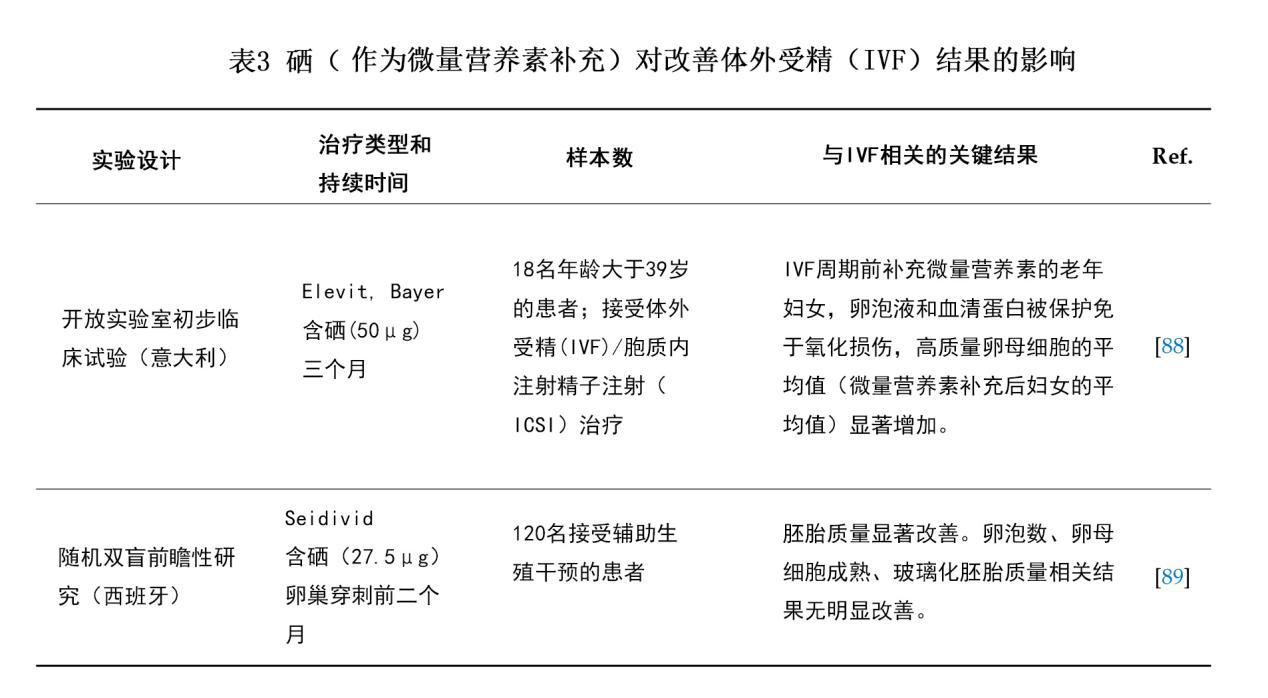
5.2 体外研究
一般来说,胚胎因比成年人更低的抗氧化酶活性从而使其对氧化损伤尤为敏感[91,92]。为了维持胚胎的生存能力与质量,一般需要特定的培养基。虽然,许多胚胎的培养环境容易实现,但要实现胚胎发育的最优环境却具一定的困难。目前,一项关于促进囊胚发育物质的研究正在进行[93,94]。为了确保所培养的细胞中的硒蛋白能正常进行生物合成,主流培养基中往往都会添加硒元素[94-96]。具有酶促活性的GPX不仅依赖于硒和维生素B6,同时也依赖于谷胱甘肽(GSH)活性[97]。猪卵母细胞中的GSH的数量不仅与受精相关,也与促进胚胎着床发育相关。胚胎期的GSH会不断减少,并在在囊胚期达到最低点。值得注意的是,在这一时期,内源性ROS的水平将达到最高[98]。由此可推断,GSH的降低与ROS的升高对正常分化过程具有一定的功能性意义[99,100]。此外,有研究发现,ROS或许对小鼠胚泡中的细胞凋亡发挥着重要调控作用[99]。
在胚胎培养基中添加硒能减少氧化损伤、调控细胞凋亡进而提高猪单性生殖胚胎的发育能力和总体质量[94]。在培养基中添加亚硒酸钠(2.5和25ng/ml)也被发现能提高囊胚率、细胞数和内细胞群比例,从而减少猪孤雌生殖胚胎中的细胞凋亡,以及BAX/BCL-xL基因比值和Caspase3的表达,并提高其GPX和ERK1/2的表达[94]。此外,补硒还能提高体外成熟卵母细胞细胞内的GPX浓度和活性,调控基因表达,改善体外受精的牛胚胎的囊胚发育和质量。近期中国的一个研究团队研究了滤泡液中的硒浓度和补硒对细胞分裂的影响,CCs中DNA的完整性,卵母细胞的发育能力,去除卵丘的卵母细胞中的GPX活性,和体外培育的牦牛卵母细胞中的硒相关基因的表达[53]。随着在体外培养基中添加硒(2和4μg/ml亚硒酸钠),与对照组(0μg/ml亚硒酸钠)相比,加硒组中所培养的卵丘细胞中的DNA损伤显著降低。同时,总GPX活性、囊胚形成率以及硒相关基因的表达都被显著上调[53]。
另一项体外研究聚焦于亚硒酸钠的作用。Abedelahi的实验组研究发现,在培养基中添加5或10ng/ml亚硒酸钠能减轻从玻璃化和非玻璃化卵巢组织中获得的窦前卵泡中由ROS引起的氧化应激,并提高其总抗氧化能力(TAC)和GPX活性[101]。此外,硒处理组的卵泡、卵母细胞和胚胎的发育率也显著上升[101]。同样的,在其早期的体外研究中,该研究团队也发现在培养基中添加5和10ng/ml亚硒酸钠将会对卵泡和卵母细胞的生长和生存能力发挥出出剂量依赖性的改善作用,这些作用或可归因于硒能以剂量依赖的方式发挥广谱抗氧化能力[102]。综上所述,这些发现可在某种程度上为促进体外卵母细胞成熟以及提高哺乳动物卵母细胞和胚胎的发育能力提供一些参考,也可作为该领域未来研究的基础。
6.母体补充膳食硒的作用
在妊娠期间,母体补充膳食硒不仅能加强抗氧化活性,促进雌二醇、孕酮和T4生成,还能提高机体对主要营养成分的新陈代谢[103]。最近几项研究评估了使用有机硒或无机硒合并其它维生素(如VB6)对母体进行营养补充的作用。结果表明,VB6对有机硒在硒依赖性GPX的抗氧化防御系统中的代谢有着重要作用[104-106]。补充膳食硒对母体的氧化应激、抗氧化活性、胚胎发育和生育能力的影响相关的一系列动物实验被总结在表4中。
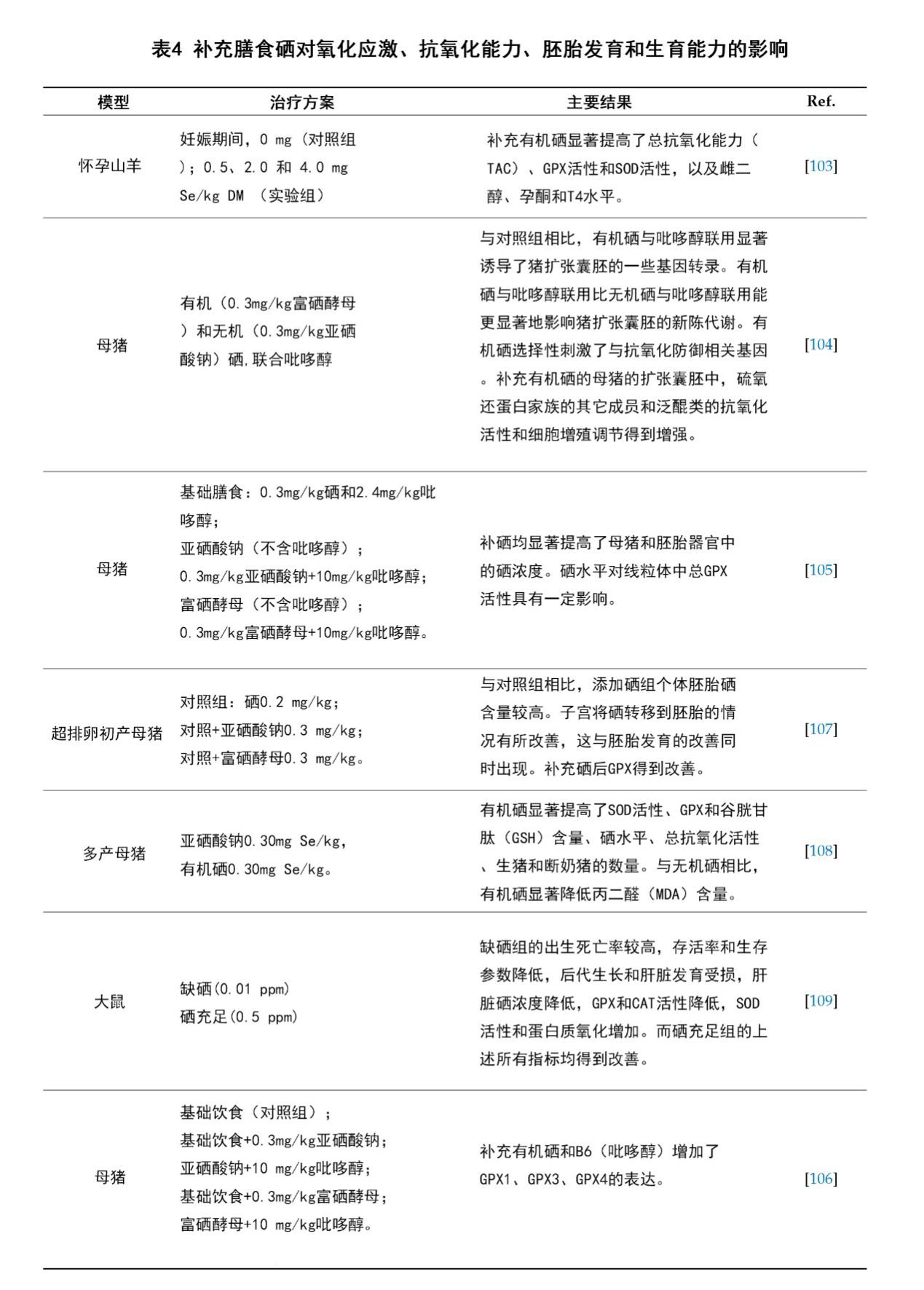
7.硒和胎盘中的氧化应激
补硒对GPX和TXNRD等内源性抗氧化酶的表达和活性是非常重要的。在细胞实验中,补硒缓解了细胞模型中的氧化应激引发的损伤,从而为微量元素补充剂在减轻某些妊娠相关疾病中的安全效用和关键机制提供了些许思路[32,110-112]。胎盘中的氧化应激对妊娠并发症(如子痫前期,子宫内生长受限,妊娠期糖尿病和早产)的病理生理和发生具有重要意义[110]。充分阐明胎盘氧化应激引起的胚胎滋养层细胞凋亡的相关机制也许能为先兆子痫的预防提供新的干预措施,据报道,先兆子痫为美国带来了每年超过8万的早产儿,在全球范围内造成了每年约七万五千名产妇的死亡[19,111]。近期一些报道揭示了硒提高整体滋养层细胞的线粒体功能和生物合成的潜在机制[32,59,110-112]。这些结果表明,硒带来的这些益处是通过提高抗氧化功能、减少ROS产物从而保护线粒体功能并增加线粒体生物合成来实现的[112]。此外,SELENOH被发现能激活NRF1和PGC-1α等转录因子来促进胚胎滋养层细胞中的线粒体生物合成[113]。这些发现有助于阐明滋养层细胞对氧化应激的响应机制,以及硒如何调控相关基因,进而通过调节线粒体功能来改善细胞存活率及其侵袭能力[114]。补硒在改善胎盘滋养层细胞系的线粒体生物合成和功能的近期相关研究汇总于表5中。
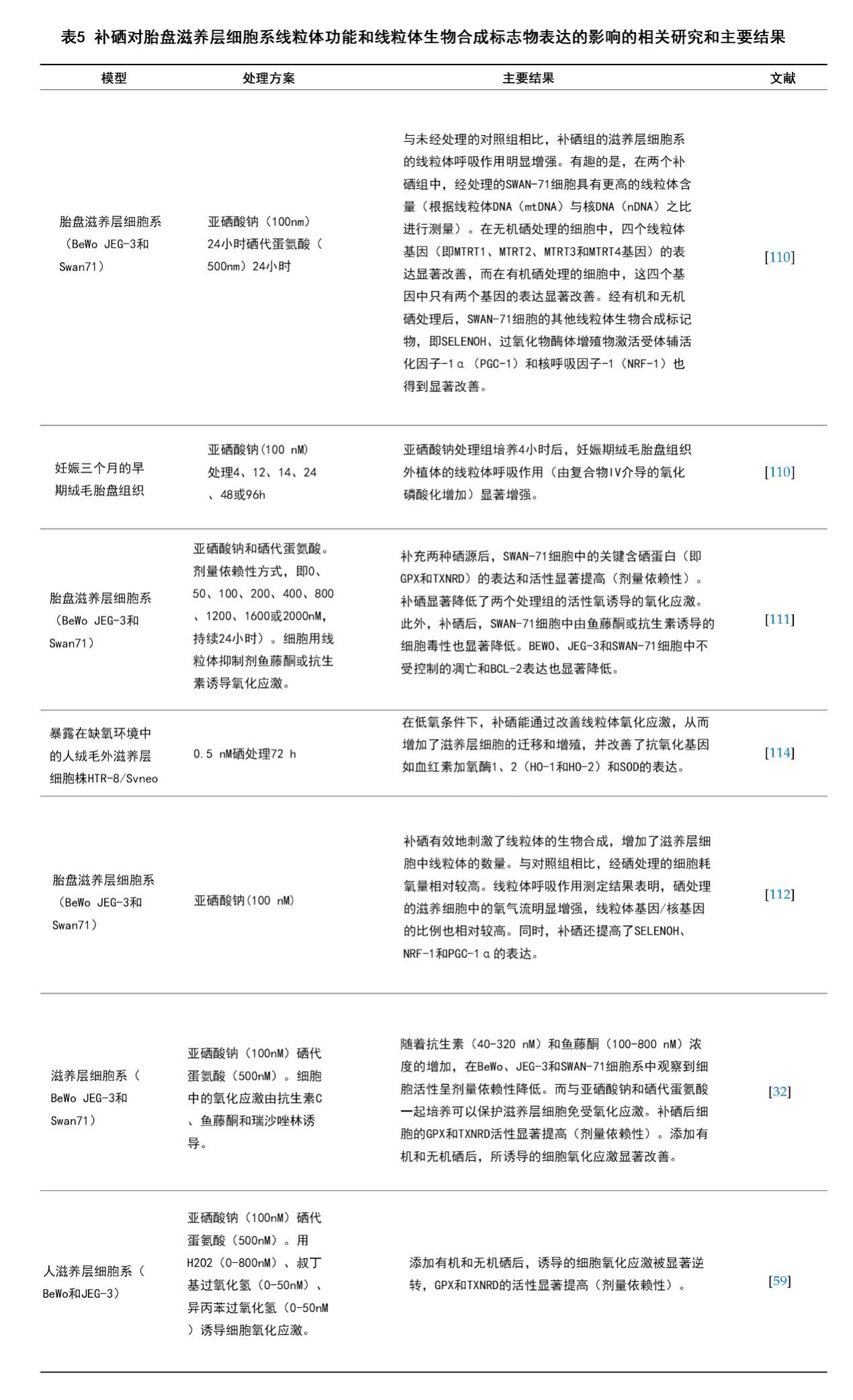
8.硒对妊娠的影响
大量研究已经证实,硒参与了GPX、SELENOP、TXNRD族等硒蛋白的生物合成,从而对妊娠有着重要作用,尤其是在妊娠期间氧化应激增加的情况下[115]。因此,抗氧化防御机制对调控氧化应激极其重要,甚至还与围产期发病率和死亡率相关[116-118]。值得注意的是,硒水平与妊娠并发症进展的关系现在仍未被充分阐明。然而,低硒水平与不良胚胎结局之间的关系已被证实。大量横截面和病例对照实验并未发现两者之间存在明显的因果关系。因此我们可以推断,妊娠并发症及随后结果可能与低硒水平有关,也可能仅仅是母体对氧化应激状态升高的简单生理反应[119]。
“妊娠前硒水平是否比妊娠期硒水平对预防妊娠高血压更重要?”这一假设目前仍需进一步验证[15]。一般来说,硒水平可能会对卵母细胞发育、受精以及着床带来影响[8,15]。因此推荐的做法是在妊娠期增加硒摄入[120]。此外,一些关于硒对生殖健康作用的研究推荐的妊娠期最佳补硒时间点差异较大。因此,迄今为止补硒的最佳时间依然没有定论[121]。与硒相关的关键信息缺乏,在很大程度上得到了其他报告的补充,这些报告鼓励进一步阐明补充微量元素的时间点对妊娠结果的潜在影响[122,123]。2017年,Mamon和Ramos进行的一项小鼠研究指出,妊娠期间补硒(3.0μg/d)的最佳时间在于围孕期的不同阶段[121]。与对照组及妊娠前-妊娠期补硒组相比,研究者们仅在妊娠前补硒组和仅在妊娠期补硒组中观察到了囊胚质量的提高和胚胎植入前的损失降低[121]。这些结果表明,妊娠前和妊娠期是围孕期补硒的两个最佳时间段,仅在其中一个时期补硒能提高囊胚的发育能力和胚胎植入的成功率[121]。
最近,一项小样本实验表明,硒与多种妊娠并发症相关[15,37,124,125]。研究发现,基线硒水平的人群的血清硒与SELENOP水平正相关[124]。此外,在正常怀孕期间,全血硒浓度因妊娠发生下降了12%[37,126,127]。血浆体积相同的情况下,妊娠期全血硒浓度下降的另一可能原因在于硒通过在胎盘中高度表达的SELENOP被转运给了胎儿[68,72]。相反,更高的硒水平则与更低风险的流产和早产相关。一些随机对照试验表明,高硒摄入或高硒水平对子痫前症和自身免疫性甲状腺疾病有益。硒能改善氧化应激、内质网应激和炎症(图1),从而保护内质网,调控类二十烷酸的生成,调控血管张力,以及抑制感染,这些对妊娠期可能都是至关重要的[29]。
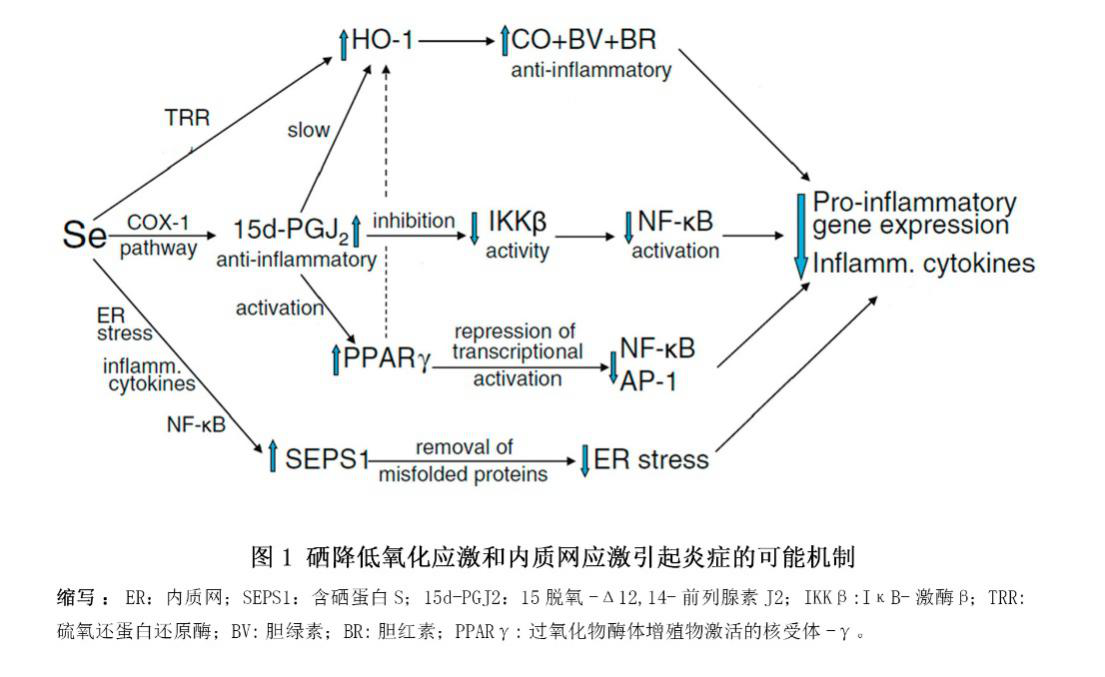
英国的一项覆盖了230名初产孕妇的双盲安慰剂对照先导实验中,将妊娠12-14周的孕妇随机分组并为其提供60μg/d富硒酵母或安慰剂直至分娩[37]。结果发现,补硒组在12-35周之间的全血硒浓度显著增加,而安慰剂组则显著降低。同样的,补硒组在妊娠35周时的全血硒和血浆SELENOP水平显著高于安慰剂组[37]。此外,该研究还发现补硒和血清可溶性血管内皮生长因子受体-1(sFlt-1)呈负相关,表明补硒有益于限制sFlt-1的生成从而具有抗血管生成的能力,并有益于改善子痫前期的病理生理。这是唯一调查并得出了“低硒水平人群补硒与子痫前期风险相关”结果的研究[37]。此外,最近一项报告显示波兰孕妇孕周硒水平逐月下降,导致大量孕妇发展成严重缺硒。妊娠期女性的平均硒和SELENOP水平也被发现在不断下降[124]。该研究发现在所有组的孕妇的三个月妊娠期中,硒与SELENOP浓度正相关[124]。相同现象出现在最近另一项关于波兰母亲与子女定群的研究中:孕妇的血浆硒浓度从第一个月到第三个月下降了23%(48.3+/-10.6到37.3+/-9.8μg/L)[125]。
子宫内生长受限是围产期死亡率的首要原因,影响了10-15%孕妇[128,129]。有报道显示,更高水平的ROS和炎症生物标记物在子宫内生长限制的发育和病理过程中起重要作用[130,131]。Mesdaghinia及其同事开展的一项随机双盲安慰剂对照试验以研究补硒对子宫内生长限制高风险孕妇(n=60)的临床症状和代谢状况的影响[131]。
硒还与其它妊娠并发症相关,如习惯性流产[56,132,133]、小于胎龄儿[119,134]和产后甲状腺功能不足[135]。一些随机对照试验和观察性研究发现,硒能减少甲状腺过氧化物酶抗体水平以及甲状腺功能减退和产后甲状腺疾病的发生[136]。甲状腺过氧化物酶抗体阳性的孕妇将会有更高风险发展成产后甲状腺功能不全(PPTD)和永久性甲状腺功能减退[135]。而硒被发现能减轻自身免疫性甲状腺炎患者的甲状腺炎[135]。一项关于甲状腺过氧化物酶抗体阳性(TPOAb阳性)孕妇的高质量随机对照试验[135]发现,与安慰剂对照组相比,每天补充200μg硒代蛋氨酸显著减少了TPOAb效价,降低了甲状腺炎、产后甲状腺疾病和永久性甲状腺功能减退的发生[135]。虽然现有文献证明补硒能以某种方式有益于减少缺硒孕妇并发症发生,然而,正在开展或未来将要开展的干预实验结果可能会加强或否定增加硒摄入的观点。
9.硒和硒蛋白对生殖系统癌症的影响
人们关于硒在癌症发生和癌症治疗方面的作用已经争论了数十年,硒蛋白会预防、抑制还是促进癌症发展目前仍争论不休[137]。卵巢癌是女性第五大癌症死因,具有最高的总体死亡率和较低的五年生存率[138]。超过90%的卵巢癌是上皮性卵巢癌(EOC),EOC中包括了病理和分子学特征不同的多种类型[138]。一些文献证据表明,硒和硒蛋白能在卵巢癌等女性生殖系统癌症中发挥作用 [139]。与健康女性相比,患有宫颈良性肿瘤及癌症、子宫体癌或卵巢癌的女性的血浆硒和GPX活性显著降低[140]。这些发现表明,患有女性生殖系统相关肿瘤的女性往往伴随着更低的抗氧化能力(与硒相关)[140]。Agnani等发现,患有乳头状浆液性卵巢癌的女性的GPX3活性呈阶段依赖性降低[139]。同时,在肿瘤复发的女性患者中的血浆GPX3水平也被观察到出现降低,且当患者和对照组只纳入50岁以上的女性时,这些水平依赖性降低变得更加明显[139]。此外,在大鼠和人类中观察到在所有级别的子宫内膜腺癌中均出现了GPX3下调[139,141]。一些早期研究发现,细胞上皮性卵巢癌组织中的GPX3高于对照组[142-144]。虽然其潜在分子学机制未被充分阐明,但这些结果已经表明GPX3活性存在肿瘤特异性[138]。在一项近期研究中,Wu及其同事发现六氟化铂敏感组中的GPX3相较于六氟化铂耐受组被下调[138]。故此可推断,GPX3抑制肿瘤的作用机制可能主要归因于启动子的超甲基化、c-Met表达的失调以及能清除有害自由基的抗氧化酶的作用[138]。然而,GPX3响应抗肿瘤药物的这种潜在机制中仍然存在大量未知,需要进一步阐明[138]。不过,硒和硒蛋白的这些作用也许能为生殖系统癌症的靶向治疗和预防提供策略。
10.总结
在本综述中,我们综合阐述了硒与硒蛋白在生殖以及一些与动物、人类生育相关疾病中的作用。从早期动物和人类研究中可看出,硒与硒蛋白能调控抗氧化平衡,因而对女性的最优生殖而言是必需的。
硒与硒蛋白在某些女性生育领域的作用目前仍未被充分阐明,需要进一步研究与思考,这些领域包括母体膳食硒摄入对氧化应激的影响,硒对胎儿卵巢发育与功能的影响,补硒对改善胎盘氧化应激的作用等。此外,人们对怀孕前时期的卵巢生理学方面,尤其是激素合成和卵巢滤泡发育方面关注较少,此外,生殖效率和生殖健康也缺乏关注。最近一些动物体外实验证据表明硒和硒蛋白在卵母细胞成熟和受精中具有潜在作用,然而体内对照试验仍然大幅度缺乏。此外,硒与硒蛋白在不同卵巢疾病(包括癌症)中作用的证据也较少且具有较大不确定性。同时,硒和硒蛋白在改善由辅助生殖技术(如IVF)和胚胎发育引起的氧化和氮化应激中的作用也未被充分阐明。另外,硒和硒蛋白与女性生殖(动物和人类)之间的关系仍存在许多问题等待人们回答。对这些问题的回答将会有助于阐明硒这种潜力非凡的微量元素对女性生育及健康的潜在生物功能。
补硒的响应曲线呈现U型,这意味着补硒虽然对缺硒个体有益,但对硒含量充足的个体或许会为其带来健康风险[145]。因此,我们必须将硒摄入量维持在合理范围,特别是对那些具有适当或高硒水平的人群。关于补硒对妊娠期的影响,一些在硒水平较低的人群中进行的高质量随机对照试验呈现出了令人振奋的结果[37]。然而,这些试验在未来仍需要纳入更多样本数以阐明硒和硒蛋白减少妊娠相关并发症(如先兆子痫)风险的潜在分子和生物化学机制。在本综述中,目前正在进行的实验和荟萃分析应该很快就能得出适当的建议。目前,向孕妇销售的含硒补充剂的使用量很大,而在发达国家,孕妇食用此类补充剂的比例非常高,这使得研究者们越来越难将受试者(孕妇)纳入随机对照试验。
综上所述,关于硒和硒蛋白在女性生殖中的改善作用的现有证据水平和质量都很高,但是这类研究的数量还不足以得出可靠的结论。因此,阐明补充硒和含硒蛋白(如GPX、SELENOP、SELENOS)对卵巢功能、妊娠相关并发症和女性整体生殖能力的潜在影响将具有巨大价值。
原文:Qazi I, Angel C, Yang H, et al. Selenium, Selenoproteins, and Female Reproduction: A Review[J]. Molecules, 2018, 23(12): 3053.
References
1. Rayman, M.P. The importance of selenium to human health. The Lancet 2000, 356, 233–241. [CrossRef]
2. Labunskyy, V.M.; Hatfield, D.L.; Gladyshev, V.N. Selenoproteins: molecular pathways and physiological roles. Physiol. Rev. 2014, 94, 739–777. [CrossRef] [PubMed]
3. Kryukov, G.V.; Castellano, S.; Novoselov, S.V.; Lobanov, A.V.; Zehtab, O.; Guigó, R.; Gladyshev, V.N. Characterization of mammalian selenoproteomes. Science 2003, 300, 1439–1443. [CrossRef] [PubMed]
4. Papp, L.V.; Lu, J.; Holmgren, A.; Khanna, K.K. From selenium to selenoproteins: synthesis, identity, and their role in human health. Antioxid. Redox Signaling 2007, 9, 775–806. [CrossRef] [PubMed]
5. Gladyshev, V.N.; Arnér, E.S.; Berry, M.J.; Brigelius-Flohé, R.; Bruford, E.A.; Burk, R.F.; Carlson, B.A.; Castellano, S.; Chavatte, L.; Conrad, M. Selenoprotein gene nomenclature. J. Biol. Chem. 2016, 291,
24036–24040. [CrossRef] [PubMed]
6. Zoidis, E.; Seremelis, I.; Kontopoulos, N.; Danezis, G.P. Selenium-Dependent Antioxidant Enzymes: Actions and Properties of Selenoproteins. Antioxidants 2018, 7, 66. [CrossRef] [PubMed] Molecules 2018, 23, 3053 18 of 24
7. Pappas, A.; Zoidis, E.; Surai, P.; Zervas, G. Selenoproteins and maternal nutrition. Comp. Biochem. Physiol. Part. B: Biochem. Mol. Biol. 2008, 151, 361–372. [CrossRef] [PubMed]
8. Ceko, M.J.; Hummitzsch, K.; Hatzirodos, N.; Bonner,W.M.; Aitken, J.B.; Russell, D.L.; Lane, M.; Rodgers, R.J.; Harris, H.H. X-Ray fluorescence imaging and other analyses identify selenium and GPX1 as important in female reproductive function. Metallomics 2015, 7, 71–82. [CrossRef] [PubMed]
9. Ceko, M.J.; Hummitzsch, K.; Bonner,W.M.; Aitken, J.B.; Spiers, K.M.; Rodgers, R.J.; Harris, H.H. Localization of the trace elements iron, zinc and selenium in relation to anatomical structures in bovine ovaries by X-ray fluorescence imaging. Microsc. Microanal. 2015, 21, 695–705. [CrossRef] [PubMed]
10. Paszkowski, T.; Traub, A.; Robinson, S.; McMaster, D. Selenium dependent glutathione peroxidase activity in human follicular fluid. Clin. Chim. Acta 1995, 236, 173–180. [CrossRef]
11. Baek, I.J.; Yon, J.M.; Lee, S.R.; Kim, M.R.; Hong, J.; Lee, B.; Yun, Y.; Nam, S.Y. Differential Expression of Gastrointestinal Glutathione Peroxidase (GI-GPx) Gene during Mouse Organogenesis. Anat. Histol. Embryo. 2011, 40, 210–218. [CrossRef] [PubMed]
12. Xu, X.; Leng, J.-Y.; Gao, F.; Zhao, Z.-A.; Deng,W.-B.; Liang, X.-H.; Zhang, Y.-J.; Zhang, Z.-R.; Li, M.; Sha, A.-G. Differential expression and anti-oxidant function of glutathione peroxidase 3 in mouse uterus during decidualization. FEBS Lett. 2014, 588, 1580–1589. [CrossRef] [PubMed]
13. Santos, C.; García-Fuentes, E.; Callejón-Leblic, B.; García-Barrera, T.; Gómez-Ariza, J.L.; Rayman, M.P.; Velasco, I. Selenium, selenoproteins and selenometabolites in mothers and babies at the time of birth. Br. J. Nutr. 2017, 117, 1304–1311. [CrossRef] [PubMed]
14. Burk, R.F.; Olson, G.E.; Hill, K.E.;Winfrey, V.P.; Motley, A.K.; Kurokawa, S. Maternal-fetal transfer of selenium in the mouse. FASEB J. 2013, 27, 3249–3256. [CrossRef] [PubMed]
15. Rayman, M.P.; Bath, S.C.;Westaway, J.;Williams, P.; Mao, J.; Vanderlelie, J.J.; Perkins, A.V.; Redman, C.W. Selenium status in UK pregnant women and its relationship with hypertensive conditions of pregnancy. Br. J. Nutr. 2015, 113, 249–258. [CrossRef] [PubMed]
16. Hayashi, K.-G.; Ushizawa, K.; Hosoe, M.; Takahashi, T. Differential genome-wide gene expression profiling of bovine largest and second-largest follicles: identification of genes associated with growth of dominant follicles. Reprod. Biol. Endocrinol. 2010, 8, 11. [CrossRef] [PubMed]
17. Yant, L.J.; Ran, Q.; Rao, L.; Van Remmen, H.; Shibatani, T.; Belter, J.G.; Motta, L.; Richardson, A.; Prolla, T.A. The selenoprotein GPX4 is essential for mouse development and protects from radiation and oxidative damage insults. Free Radical Biol. Med. 2003, 34, 496–502. [CrossRef]
18. Imai, H.; Hirao, F.; Sakamoto, T.; Sekine, K.; Mizukura, Y.; Saito, M.; Kitamoto, T.; Hayasaka, M.; Hanaoka, K.; Nakagawa, Y. Early embryonic lethality caused by targeted disruption of the mouse PHGPx gene. Biochem. Biophys. Res. Commun. 2003, 305, 278–286. [CrossRef]
19. Mistry, H.D.;Wilson, V.; Ramsay, M.M.; Symonds, M.E.; Pipkin, F.B. Reduced selenium concentrations and glutathione peroxidase activity in preeclamptic pregnancies. Hypertension 2008, 52, 881–888. [CrossRef]
[PubMed]
20. Peng, X.; Lin, Y.; Li, J.; Liu, M.;Wang, J.; Li, X.; Liu, J.; Jia, X.; Jing, Z.; Huang, Z. Evaluation of Glutathione Peroxidase 4 role in Preeclampsia. Sci. Rep. 2016, 6, 33300. [CrossRef] [PubMed]
21. Malinova, M.; Paskaleva, V. Selenium and glutathione peroxidase in patients with preeclampsia. Akush. Ginekol. 2013, 52, 3–7.
22. Imai, H.; Hakkaku, N.; Iwamoto, R.; Suzuki, J.; Suzuki, T.; Tajima, Y.; Konishi, K.; Minami, S.; Ichinose, S. Ishizaka, K. Depletion of selenoprotein GPx4 in spermatocytes causes male infertility in mice. J. Biol. Chem. 2009, 284, 32522–32532. [CrossRef] [PubMed]
23. Ahsan, U.; Kamran, Z.; Raza, I.; Ahmad, S.; Babar, W.; Riaz, M.; Iqbal, Z. Role of selenium in male reproduction—A review. Anim. Reprod. Sci. 2014, 146, 55–62. [CrossRef] [PubMed]
24. Chabory, E.; Damon, C.; Lenoir, A.; Kauselmann, G.; Kern, H.; Zevnik, B.; Garrel, C.; Saez, F.; Cadet, R.; Henry-Berger, J. Epididymis seleno-independent glutathione peroxidase 5 maintains sperm DNA integrity in mice. J. Clin. Investing. 2009, 119, 2074–2085. [CrossRef] [PubMed]
25. Jakupoglu, C.; Przemeck, G.K.; Schneider, M.; Moreno, S.G.; Mayr, N.; Hatzopoulos, A.K.; de Angelis, M.H.; Wurst,W.; Bornkamm, G.W.; Brielmeier, M. Cytoplasmic thioredoxin reductase is essential for embryogenesis but dispensable for cardiac development. Mol. Cell. Biol. 2005, 25, 1980–1988. [CrossRef] [PubMed] Molecules 2018, 23, 3053 19 of 24
26. Bondareva, A.A.; Capecchi, M.R.; Iverson, S.V.; Li, Y.; Lopez, N.I.; Lucas, O.; Merrill, G.F.; Prigge, J.R.; Siders, A.M.;Wakamiya, M. Effects of thioredoxin reductase-1 deletion on embryogenesis and transcriptome. Free Radical Biol. Med. 2007, 43, 911–923. [CrossRef] [PubMed]
27. Conrad, M.; Jakupoglu, C.; Moreno, S.G.; Lippl, S.; Banjac, A.; Schneider, M.; Beck, H.; Hatzopoulos, A.K.; Just, U.; Sinowatz, F. Essential role for mitochondrial thioredoxin reductase in hematopoiesis, heart development, and heart function. Mol. Cell. Biol. 2004, 24, 9414–9423. [CrossRef] [PubMed]
28. Su, D.; Novoselov, S.V.; Sun, Q.-A.; Moustafa, M.E.; Zhou, Y.; Oko, R.; Hatfield, D.L.; Gladyshev, V.N. Mammalian selenoprotein thioredoxin-glutathione reductase roles in disulfide bond formation and sperm maturation. J. Biol. Chem. 2005, 280, 26491–26498. [CrossRef] [PubMed]
29. Rayman, M.P. Selenium and adverse health conditions of human pregnancy. In Selenium; Springer: New York, NY, USA, 2011; pp. 531–544.
30. Galton, V.A.; Martinez, E.; Hernandez, A.; Germain, E.A.S.; Bates, J.M.; Germain, D.L.S. Pregnant rat uterus expresses high levels of the type 3 iodothyronine deiodinase. J. Clin. Investing. 1999, 103, 979–987. [CrossRef] [PubMed]
31. Huang, S.A.; Dorfman, D.M.; Genest, D.R.; Salvatore, D.; Larsen, P.R. Type 3 iodothyronine deiodinase is highly expressed in the human uteroplacental unit and in fetal epithelium. J. Clin. Endocrinol. Metab. 2003, 88, 1384–1388. [CrossRef] [PubMed]
32. Khera, A.; Vanderlelie, J.; Perkins, A. Selenium supplementation protects trophoblast cells from mitochondrial oxidative stress. Placenta 2013, 34, 594–598. [CrossRef] [PubMed]
33. Michaelis, M.; Gralla, O.; Behrends, T.; Scharpf, M.; Endermann, T.; Rijntjes, E.; Pietschmann, N.; Hollenbach, B.; Schomburg, L. Selenoprotein P in seminal fluid is a novel biomarker of sperm quality. Biochem. Biophys. Res. Commun. 2014, 443, 905–910. [CrossRef] [PubMed]
34. Renko, K.;Werner, M.; Renner-Muller, I.; Cooper, T.G.; Yeung, C.H.; Hollenbach, B.; Scharpf, M.; Kohrle, J.; Schomburg, L.; Schweizer, U. Hepatic selenoprotein P (SePP) expression restores selenium transport and prevents infertility and motor-incoordination in Sepp-knockout mice. Biochem. J. 2008, 409, 741–749. [CrossRef] [PubMed]
35. Olson, G.E.;Winfrey, V.P.; NagDas, S.K.; Hill, K.E.; Burk, R.F. Apolipoprotein E receptor-2 (ApoER2) mediates selenium uptake from selenoprotein P by the mouse testis. J. Biol. Chem. 2007, 282, 12290–12297. [CrossRef] [PubMed]
36. Olson, G.E.;Winfrey, V.P.; NagDas, S.K.; Hill, K.E.; Burk, R.F. Selenoprotein P is required for mouse sperm development. Biol. Reprod. 2005, 73, 201–211. [CrossRef] [PubMed]
37. Rayman, M.P.; Searle, E.; Kelly, L.; Johnsen, S.; Bodman-Smith, K.; Bath, S.C.; Mao, J.; Redman, C.W. Effect of selenium on markers of risk of pre-eclampsia in UK pregnant women: a randomised, controlled pilot trial. Br. J. Nutr. 2014, 112, 99–111. [CrossRef] [PubMed]
38. Moses, E.K.; Johnson, M.P.; Tommerdal, L.; Forsmo, S.; Curran, J.E.; Abraham, L.J.; Charlesworth, J.C.; Brennecke, S.P.; Blangero, J.; Austgulen, R. Genetic association of preeclampsia to the inflammatory response gene SEPS1. Am. J. Obstet. Gynecol. 2008, 198, 20. [CrossRef] [PubMed]
39. Turanov, A.A.; Malinouski, M.; Gladyshev, V.N. Selenium and male reproduction. In Selenium: Its Molecular Biology and Role in Human Health; Hatfield, D., Berry, M., Gladyshev, V., Eds.; Springer: New York, NY, USA, 2011; pp. 409–417.
40. Letavayová, L.; Vlˇcková, V.; Brozmanová, J. Selenium: from cancer prevention to DNA damage. Toxicology 2006, 227, 1–14. [CrossRef] [PubMed]
41. Schrauzer, G.N. Selenomethionine: A review of its nutritional significance, metabolism and toxicity. J. Nutr. 2000, 130, 1653–1656. [CrossRef] [PubMed]
42. Rayman, M.P.; Infante, H.G.; Sargent, M. Food-chain selenium and human health: spotlight on speciation. Br. J. Nutr. 2008, 100, 238–253. [CrossRef] [PubMed]
43. Institute of Medicine: Panel on Dietary Antioxidants and Related Compounds: Dietary Reference Intakes for Vitamin C, Vitamin E, Selenium, and Carotenoids. Washington (DC). Available online: https://www.ncbi. nlm.nih.gov/books/NBK225470/ (accessed on 19 November 2018).
44. Council, N.R. Nutrient Requirements of Small Ruminants: Sheep, Goats, Cervids, and New World Camelids; The National Academies Press: Washington, DC, USA, 2007; p. 384.
45. Surai, P.F.; Fisinin, V.I. Selenium in Pig Nutrition and reproduction: Boars and semen quality—A Review. Asian-Australas. J. Anim. Sci. 2015, 28, 730. [CrossRef] [PubMed] Molecules 2018, 23, 3053 20 of 24
46. Council, N.R. Nutrient Requirements of Horses: Sixth Revised Edition; The National Academies Press: Washington, DC, USA, 2007; p. 360.
47. Geor, R.J.; Coenen, M.; Harris, P. Equine Applied and Clinical Nutrition E-Book: Health, Welfare and Performance. FEMS Microbiol. Lett. 2014, 57, 73–77.
48. Council, N.R. Nutrient Requirements of Dairy Cattle: Seventh Revised Edition, 2001; The National Academies Press: Washington, DC, USA, 2001; p. 408.
49. Council, N.R. Nutrient Requirements of Beef Cattle: Seventh Revised Edition: Update 2000; The National Academies Press: Washington, DC, USA, 2000; p. 248.
50. Faye, B.; Seboussi, R. Selenium in camel–A review. Nutrients 2009, 1, 30–49. [CrossRef] [PubMed]
51. Rayman, M.P. The use of high-selenium yeast to raise selenium status: how does it measure up? Br. J. Nutr. 2004, 92, 557–573. [CrossRef] [PubMed]
52. Foresta, C.; Flohé, L.; Garolla, A.; Roveri, A.; Ursini, F.; Maiorino, M. Male fertility is linked to the selenoprotein phospholipid hydroperoxide glutathione peroxidase. Biol. Reprod. 2002, 67, 967–971. [CrossRef] [PubMed]
53. Xiong, X.; Lan, D.; Li, J.; Lin, Y.; Li, M. Selenium supplementation during in vitro maturation enhances meiosis and developmental capacity of yak oocytes. Anim. Sci. J. 2018, 89, 298–306. [CrossRef] [PubMed]
54. Kommisrud, E.; Østerås, O.; Vatn, T. Blood selenium associated with health and fertility in Norwegian dairy herds. Acta Vet. Scand. 2005, 46, 229. [CrossRef] [PubMed]
55. Mehdi, Y.; Dufrasne, I. Selenium in cattle: a review. Molecules 2016, 21, 545. [CrossRef] [PubMed]
56. Al-Kunani, A.; Knight, R.; Haswell, S.; Thompson, J.; Lindow, S. The selenium status of women with a history of recurrent miscarriage. BJOG: An. Int. J. Obstet. Gynaecol. 2001, 108, 1094–1097.
57. Güvenç, M.; Güven, H.; Karata¸s, F.; Aygün, A.D.; Bekta¸s, S. Low levels of selenium in miscarriage. J. Trace Elements Exp. Med. 2002, 15, 97–101. [CrossRef]
58. Rumiris, D.; Purwosunu, Y.; Wibowo, N.; Farina, A.; Sekizawa, A. Lower rate of preeclampsia after antioxidant supplementation in pregnant women with low antioxidant status. Hypertens. Pregnancy 2006, 25, 241–253. [CrossRef] [PubMed]
59. Watson, M.; Van Leer, L.; Vanderlelie, J.; Perkins, A. Selenium supplementation protects trophoblast cells from oxidative stress. Placenta 2012, 33, 1012–1019. [CrossRef] [PubMed]
60. Tsuzuki, S.; Morimoto, N.; Hosokawa, S.; Matsushita, T. Associations of maternal and neonatal serum trace element concentrations with neonatal birth weight. PLoS ONE 2013, 8, e75627. [CrossRef] [PubMed]
61. Yao, X.; Ei-Samahy, M.; Fan, L.; Zheng, L.; Jin, Y.; Zhang, G.; Liu, Z.;Wang, F. In vitro influence of selenium on the proliferation of and steroidogenesis in goat luteinized granulosa cells. Theriogenology 2018, 114, 70–80. [CrossRef] [PubMed]
62. Hill, K.E.; Lyons, P.R.; Burk, R.F. Differential regulation of rat liver selenoprotein mRNAs in selenium deficiency. Biochem. Biophys Res. Commun. 1992, 185, 260–263. [CrossRef]
63. Bösl, M.R.; Takaku, K.; Oshima, M.; Nishimura, S.; Taketo, M.M. Early embryonic lethality caused by targeted disruption of the mouse selenocysteine tRNA gene (Trsp). Proc. Natl. Acad. Sci. 1997, 94, 5531–5534. [CrossRef] [PubMed]
64. Lee, A.M.; Huel, G.; Godin, J.; Hellier, G.; Sahuquillo, J.; Moreau, T.; Blot, P. Inter-individual variation of selenium in maternal plasma, cord plasma and placenta. Sci. Total Environ. 1995, 159, 119–127. [CrossRef]
65. Eisenmann, C.; Miller, R. The placental transfer and toxicity of selenite relative to cadmium in the human term perfused placenta. Placenta 1994, 15, 883–895. [CrossRef]
66. Olson, G.E.; Whitin, J.C.; Hill, K.E.; Winfrey, V.P.; Motley, A.K.; Austin, L.M.; Deal, J.; Cohen, H.J.; Burk, R.F. Extracellular glutathione peroxidase (Gpx3) binds specifically to basement membranes of mouse renal cortex tubule cells. Am. J. Physiol. Ren. Physiol. 2009, 298, F1244–F1253. [CrossRef] [PubMed]
67. Burk, R.F.; Hill, K.E. Selenoprotein P—expression, functions, and roles in mammals. Biochim. et Biophys. Acta Gen. Subj. 2009, 1790, 1441–1447. [CrossRef] [PubMed]
68. Burk, R.F.; Hill, K.E. Regulation of selenium metabolism and transport. Annu. Rev. Nutr. 2015, 35, 109–134. [CrossRef] [PubMed]
69. Kurokawa, S.; Hill, K.E.; McDonald, W.H.; Burk, R.F. Long-isoform mouse selenoprotein P (Sepp1) supplies rat myoblast L8 cells with selenium via endocytosis mediated by heparin-binding properties and apolipoprotein E receptor-2 (apoER2). J. Biol. Chem. 2012, 287, 28717–28726. [CrossRef] [PubMed] Molecules 2018, 23, 3053 21 of 24
70. Bou-Resli, M.; Dashti, H.; Mathew, T.; Al-Zaid, N. Pre-and postnatal tissue selenium of the rat in the growing state. Neonatology 2001, 80, 169–172. [CrossRef] [PubMed]
71. Hatzirodos, N.; Hummitzsch, K.; Irving-Rodgers, H.F.; Harland, M.L.; Morris, S.E.; Rodgers, R.J. Transcriptome profiling of granulosa cells from bovine ovarian follicles during atresia. BMC Genomics 2014, 15, 40. [CrossRef] [PubMed]
72. Kasik, J.; Rice, E. Selenoprotein P expression in liver, uterus and placenta during late pregnancy. Placenta 1995, 16, 67–74. [CrossRef]
73. Galton, V.A.; Martinez, E.; Hernandez, A.; St. Germain, E.A.; Bates, J.M.; St. Germain, D.L. The type 2 iodothyronine deiodinase is expressed in the rat uterus and induced during pregnancy. Endocrinology 2001, 142, 2123–2128. [CrossRef] [PubMed]
74. Koopdonk-Kool, J.M.; De Vijlder, J.; Veenboer, G.J.; Ris-Stalpers, C.; Kok, J.H.; Vulsma, T.; Boer, K.; Visser, T.J. Type II and type III deiodinase activity in human placenta as a function of gestational age. J. Clin. Endocrinol. Metab. 1996, 81, 2154–2158. [PubMed]
75. Ejima, K.; Koji, T.; Nanri, H.; Kashimura, M.; Ikeda, M. Expression of thioredoxin and thioredoxin reductase in placentae of pregnant mice exposed to lipopolysaccharide. Placenta 1999, 20, 561–566. [CrossRef] [PubMed]
76. Ejima, K.; Nanri, H.; Toki, N.; Kashimura, M.; Ikeda, M. Localization of thioredoxin reductase and thioredoxin in normal human placenta and their protective effect against oxidative stress. Placenta 1999, 20, 95–101. [CrossRef] [PubMed]
77. Basini, G.; Tamanini, C. Selenium stimulates estradiol production in bovine granulosa cells: possible involvement of nitric oxide. Domes. Anim. Endocrinol. 2000, 18, 1–17. [CrossRef]
78. Grazul-Bilska, A.T.; Caton, J.S.; Arndt, W.; Burchill, K.; Thorson, C.; Borowczyk, E.; Bilski, J.J.; Redmer, D.A.; Reynolds, L.P.; Vonnahme, K.A. Cellular proliferation and vascularization in ovine fetal ovaries: Effects of undernutrition and selenium in maternal diet. Reproduction 2009, 137, 699–707. [CrossRef] [PubMed]
79. Kamada, H.; Ikumo, H. Effect of selenium on cultured bovine luteal cells. Anim. Reprod. Sci. 1997, 46, 203–211. [CrossRef]
80. Tilly, J.L.; Tilly, K. Inhibitors of oxidative stress mimic the ability of follicle-stimulating hormone to suppress apoptosis in cultured rat ovarian follicles. Endocrinology 1995, 136, 242–252. [CrossRef] [PubMed]
81. Grabek, M.; Swies, Z.; Borzecki, A. The influence of selenium on the reproduction of rats. Eur. PMC 1991, 46, 103–105.
82. Özkaya, M.O.; Naz?ro? glu, M.; Barak, C.; Berkkanoglu, M. Effects of multivitamin/mineral supplementation on trace element levels in serum and follicular fluid of women undergoing in vitro fertilization (IVF). Biol. Trace Elem. Res. 2011, 139, 1–9. [CrossRef] [PubMed]
83. Ruder, E.H.; Hartman, T.J.; Goldman, M.B. Impact of oxidative stress on female fertility. Curr. Opin. Obstet. Gynecol. 2009, 21, 219–222. [CrossRef] [PubMed]
84. Mehdi, Y.; Hornick, J.-L.; Istasse, L.; Dufrasne, I. Selenium in the environment, metabolism and involvement in body functions. Molecules 2013, 18, 3292–3311. [CrossRef] [PubMed]
85. Edassery, S.L.; Shatavi, S.V.; Kunkel, J.P.; Hauer, C.; Brucker, C.; Penumatsa, K.; Yu, Y.; Dias, J.A.; Luborsky, J.L. Autoantigens in ovarian autoimmunity associated with unexplained infertility and premature ovarian failure. Fertil. Steril. 2010, 94, 2636–2641. [CrossRef] [PubMed]
86. Singh, A.K.; Chattopadhyay, R.; Chakravarty, B.; Chaudhury, K. Markers of oxidative stress in follicular fluid of women with endometriosis and tubal infertility undergoing IVF. Reprod. Toxicol. 2013, 42, 116–124. [CrossRef] [PubMed]
87. Coskun, A.; Arikan, T.; Kilinc, M.; Arikan, D.C.; Ekerbiçer, H.Ç. Plasma selenium levels in Turkish women with polycystic ovary syndrome. Eur. J. Obstet. Gynecol. Reprod. Biol. 2013, 168, 183–186. [CrossRef] [PubMed]
88. Luddi, A.; Capaldo, A.; Focarelli, R.; Gori, M.; Morgante, G.; Piomboni, P.; De Leo, V. Antioxidants reduce oxidative stress in follicular fluid of aged women undergoing IVF. Reprod. Biol. Endocrinol. 2016, 14, 57. [CrossRef] [PubMed]
89. Jiménez Tuñón, J.M.; Trilles, P.P.; Molina, M.G.; Duvison, M.H.; Pastor, B.M.; Martín, P.S.; Martín, F.S.; Sánchez-Borrego, R. A Double-Blind, Randomized Prospective Study to Evaluate the Efficacy of Previous TherapyWith Melatonin, Myo-inositol, Folic Acid, and Selenium in Improving the Results of an Assisted Reproductive Treatment. Clin. Med. Insight. Ther. 2017, 9, 1179559X17742902. [CrossRef] Molecules 2018, 23, 3053 22 of 24
90. Wilson, R.L.; Bianco-Miotto, T.; Leemaqz, S.Y.; Grzeskowiak, L.E.; Dekker, G.A.; Roberts, C.T. Early pregnancy maternal trace mineral status and the association with adverse pregnancy outcome in a cohort of Australian women. J. Trace Elem. Med. Biol. 2018, 46, 103–109. [CrossRef] [PubMed]
91. Parman, T.; Wiley, M.J.; Wells, P.G. Free radical-mediated oxidative DNA damage in the mechanism of thalidomide teratogenicity. Nat. Med. 1999, 5, 582–585. [CrossRef] [PubMed]
92. Winn, L.M.; Wells, P.G. Maternal administration of superoxide dismutase and catalase in phenytoin teratogenicity1. Free Radical Biol. Med. 1999, 26, 266–274. [CrossRef]
93. Thompson, J. In vitro culture and embryo metabolism of cattle and sheep embryos—a decade of achievement. Anim. Reprod. Sci. 2000, 60, 263–275. [CrossRef]
94. Uhm, S.J.; Gupta, M.K.; Yang, J.H.; Lee, S.H.; Lee, H.T. Selenium improves the developmental ability and reduces the apoptosis in porcine parthenotes. Mol. Reprod. Dev. 2007, 74, 1386–1394. [CrossRef] [PubMed]
95. McKeehan, W.L.; Hamilton, W.G.; Ham, R.G. Selenium is an essential trace nutrient for growth of WI-38 diploid human fibroblasts. Proc. Natl. Acad. Sci. 1976, 73, 2023–2027. [CrossRef] [PubMed]
96. Hewlett, G. Strategies for optimising serum-free media. Cytotechnology 1991, 5, 3–14. [CrossRef] [PubMed]
97. Ufer, C.; Wang, C.C. The roles of glutathione peroxidases during embryo development. Front. Mol. Neurosci. 2011, 4, 12. [CrossRef] [PubMed]
98. Yoshida, M.; Ishigaki, K.; Nagai, T.; Chikyu, M.; Pursel, V.G. Glutathione concentration during maturation and after fertilization in pig oocytes: relevance to the ability of oocytes to form male pronucleus. Biol. Reprod. 1993, 49, 89–94. [CrossRef] [PubMed]
99. Pierce, G.B.; Parchment, R.E.; Lewellyn, A.L. Hydrogen peroxide as a mediator of programmed cell death in the blastocyst. Differentiation 1991, 46, 181–186. [CrossRef] [PubMed]
100. Parchment, R. The implications of a unified theory of programmed cell death, polyamines, oxyradicals and histogenesis in the embryo. Int. J. Dev. Biol. 2003, 37, 75–83.
101. Abedelahi, A.; Salehnia, M.; Allameh, A.; Davoodi, D. Sodium selenite improves the in vitro follicular development by reducing the reactive oxygen species level and increasing the total antioxidant capacity and glutathione peroxide activity. Hum. Reprod. 2010, 25, 977–985. [CrossRef] [PubMed]
102. Abedelahi, A.; Salehnia, M.; Allameh, A. The effects of different concentrations of sodium selenite on the in vitro maturation of preantral follicles in serum-free and serum supplemented media. J. Assist. Reprod. Genet. 2008, 25, 483–488. [CrossRef] [PubMed]
103. Shi, L.; Ren, Y.; Zhang, C.; Yue, W.; Lei, F. Effects of organic selenium (Se-enriched yeast) supplementation in gestation diet on antioxidant status, hormone profile and haemato-biochemical parameters in Taihang Black Goats. Anim. Feed Sci. Technol. 2018, 238, 57–65. [CrossRef]
104. Dalto, B.; Tsoi, S.; Audet, I.; Dyck, M.K.; Foxcroft, G.; Matte, J.J. Gene expression of porcine blastocysts from gilts fed organic or inorganic selenium and pyridoxine. Reproduction 2015, 149, 31–42. [CrossRef] [PubMed]
105. Dalto, D.B.; Audet, I.; Lapointe, J.; Matte, J.J. The importance of pyridoxine for the impact of the dietary selenium sources on redox balance, embryo development, and reproductive performance in gilts. J. Trace Elem. Med. Biol. 2016, 34, 79–89. [CrossRef] [PubMed]
106. Dalto, D.B.; Roy, M.; Audet, I.; Palin, M.-F.; Guay, F.; Lapointe, J.; Matte, J.J. Interaction between vitamin B6 and source of selenium on the response of the selenium-dependent glutathione peroxidase system to oxidative stress induced by oestrus in pubertal pig. J. Trace Elem. Med. Biol. 2015, 32, 21–29. [CrossRef] [PubMed]
107. Fortier, M.E.; Audet, I.; Giguere, A.; Laforest, J.P.; Bilodeau, J.F.; Quesnel, H.; Matte, J.J. Effect of dietary organic and inorganic selenium on antioxidant status, embryo development, and reproductive performance in hyperovulatory first-parity gilts. J. Anim. Sci. 2012, 90, 231–240. [CrossRef] [PubMed]
108. Chen, J.; Han, J.; Guan, W.; Chen, F.; Wang, C.; Zhang, Y.; Lv, Y.; Lin, G. Selenium and vitamin E in sow diets: I. Effect on antioxidant status and reproductive performance in multiparous sows. Anim. Feed Sci. Technol. 2016, 221, 111–123. [CrossRef]
109. Nogales, F.; Ojeda, M.L.; Fenutría, M.; Murillo, M.L.; Carreras, O. Role of selenium and glutathione peroxidase on development, growth, and oxidative balance in rat offspring. Reproduction 2013, 146, 659–667. [CrossRef] [PubMed]
110. Khera, A.; Dong, L.-F.; Holland, O.; Vanderlelie, J.; Pasdar, E.A.; Neuzil, J.; Perkins, A.V. Selenium supplementation induces mitochondrial biogenesis in trophoblasts. Placenta 2015, 36, 863–869. [CrossRef] [PubMed] Molecules 2018, 23, 3053 23 of 24
111. Khera, A.; Vanderlelie, J.J.; Holland, O.; Perkins, A.V. Overexpression of endogenous anti-oxidants with selenium supplementation protects trophoblast cells from reactive oxygen species-induced apoptosis in a Bcl-2-dependent manner. Biol. Trace Elem. Res. 2017, 177, 394–403. [CrossRef] [PubMed]
112. Perkins, A.; Khera, A.; Holland, O.; Vanderlelie, J. Trophoblast mitochondrial biogenesis and functionality is increased with selenium supplementation. Placenta 2016, 45, 118. [CrossRef]
113. Mendelev, N.; Mehta, S.L.; Idris, H.; Kumari, S.; Li, P.A. Selenite stimulates mitochondrial biogenesis signaling and enhances mitochondrial functional performance in murine hippocampal neuronal cells. PLoS ONE 2012, 7, e47910. [CrossRef] [PubMed]
114. Na, J.Y.; Seok, J.; Park, S.; Kim, J.S.; Kim, G.J. Effects of selenium on the survival and invasion of trophoblasts. Clin. Exp. Reprod. Med. 2018, 45, 10–16. [CrossRef] [PubMed]
115. Fialova, L.; Malbohan, I.; Kalousova, M.; Soukupova, J.; Krofta, L.; Štipek, S.; Zima, T. Oxidative stress and inflammation in pregnancy. Scand. J. Clin. Lab. INVEST. 2006, 66, 121–128. [CrossRef] [PubMed]
116. Orhan, H.; Önderoglu, L.; Yücel, A.; Sahin, G. Circulating biomarkers of oxidative stress in complicated pregnancies. Arch. Gynecol. Obst. 2003, 267, 189–195.
117. Karowicz-Bilinska, A.; K?edziora-Kornatowska, K.; Bartosz, G. Indices of oxidative stress in pregnancy with fetal growth restriction. Free Radical Res. 2007, 41, 870–873. [CrossRef] [PubMed]
118. Hracsko, Z.; Orvos, H.; Novak, Z.; Pal, A.; Varga, I.S. Evaluation of oxidative stress markers in neonates with intra-uterine growth retardation. Redox Rep. 2008, 13, 11–16. [CrossRef] [PubMed]
119. Mariath, A.B.; Bergamaschi, D.P.; Rondó, P.H.; Ana, C.A.T.; de Fragas Hinnig, P.; Abbade, J.F.; Diniz, S.G. The possible role of selenium status in adverse pregnancy outcomes. Br. J. Nutr. 2011, 105, 1418–1428. [CrossRef] [PubMed]
120. Richard, K.; Holland, O.; Landers, K.; Vanderlelie, J.J.; Hofstee, P.; Cuffe, J.S.; Perkins, A.V. Effects of maternal micronutrient supplementation on placental function. Placenta 2017, 54, 38–44. [CrossRef] [PubMed]
121. Mamon, M.A.C.; Ramos, G.B. Maternal selenium-supplementation at various stages of periconception period: influence on murine blastocyst morphology and implantation status. J. Anim. Sci. Technol. 2017, 59, 7. [CrossRef] [PubMed]
122. Cetin, I.; Berti, C.; Calabrese, S. Role of micronutrients in the periconceptional period. Hum. Reprod. Update 2009, 16, 80–95. [CrossRef] [PubMed]
123. Berti, C.; Biesalski, H.; Gärtner, R.; Lapillonne, A.; Pietrzik, K.; Poston, L.; Redman, C.; Koletzko, B.; Cetin, I. Micronutrients in pregnancy: current knowledge and unresolved questions. Clin. Nutr. 2011, 30, 689–701. [CrossRef] [PubMed]
124. Ambroziak, U.; Hybsier, S.; Shahnazaryan, U.; Krasnod?ebska-Kilja ´ nska, M.; Rijntjes, E.; Bartoszewicz, Z.; Bednarczuk, T.; Schomburg, L. Severe selenium deficits in pregnant women irrespective of autoimmune thyroid disease in an area with marginal selenium intake. J. Trace Elem. Med. Biol. 2017, 44, 186–191. [CrossRef] [PubMed]
125. Polanska, K.; Krol, A.; Sobala,W.; Gromadzinska, J.; Brodzka, R.; Calamandrei, G.; Chiarotti, F.;Wasowicz,W.; Hanke, W. Selenium status during pregnancy and child psychomotor development—Polish Mother and Child Cohort study. Pediatr. Res. 2016, 79, 863. [CrossRef] [PubMed]
126. Butler, J.A.; Whanger, P.D.; Tripp, M.J. Blood selenium and glutathione peroxidase activity in pregnant women: comparative assays in primates and other animals. Am. J. Clin. Nutr. 19821982, 36, 15–23. [CrossRef] [PubMed]
127. Zachara, B.; Wardak, C.; Didkowski, W.; Maciag, A.; Marchaluk, E. Changes in blood selenium and glutathione concentrations and glutathione peroxidase activity in human pregnancy. Gynecol. Obst. Invest. 1993, 35, 12–17. [CrossRef] [PubMed]
128. Jarvis, S.; Glinianaia, S.V.; Torrioli, M.-G.; Platt, M.-J.; Miceli, M.; Jouk, P.-S.; Johnson, A.; Hutton, J.; Hemming, K.; Hagberg, G. Cerebral palsy and intrauterine growth in single births: European collaborative study. The Lancet 2003, 362, 1106–1111. [CrossRef]
129. Dikbas, L.; Yapca, O.E.; Dikbas, N.; Gundogdu, C. Paraoxonase-2 and paraoxonase-3: comparison of mRNA expressions in the placentae of unexplained intrauterine growth restricted and noncomplicated pregnancies. J. Mat.-Fet. Neonatal Med. 2017, 30, 1200–1206. [CrossRef] [PubMed]
130. Takagi, Y.; Nikaido, T.; Toki, T.; Kita, N.; Kanai, M.; Ashida, T.; Ohira, S.; Konishi, I. Levels of oxidative stress and redox-related molecules in the placenta in preeclampsia and fetal growth restriction. Virchows Arch. 2004, 444, 49–55. [CrossRef] [PubMed] Molecules 2018, 23, 3053 24 of 24
131. Mesdaghinia, E.; Rahavi, A.; Bahmani, F.; Sharifi, N.; Asemi, Z. Clinical and Metabolic Response to Selenium Supplementation in PregnantWomen at Risk for Intrauterine Growth Restriction: Randomized, Double-Blind, Placebo-Controlled Trial. Biol. Trace Elem. Res. 2017, 178, 14–21. [CrossRef] [PubMed]
132. Barrington, J.; Lindsay, P.; James, D.; Smith, S.; Roberts, A. Selenium deficiency and miscarriage: A possible link? Int. J. Obstet. Gynaecol. 1996, 103, 130–132. [CrossRef]
133. Koçak, ˙I.; Aksoy, E.; Üstün, C. Recurrent spontaneous abortion and selenium deficiency. Int. J. Obstet. Gynaecol. 1999, 65, 79–80. [CrossRef]
134. Mistry, H.D.; Kurlak, L.O.; Young, S.D.; Briley, A.L.; Broughton Pipkin, F.; Baker, P.N.; Poston, L. Maternal selenium, copper and zinc concentrations in pregnancy associated with small-for-gestational-age infants. Mat. Child Nutr. 2014, 10, 327–334. [CrossRef] [PubMed]
135. Negro, R.; Greco, G.; Mangieri, T.; Pezzarossa, A.; Dazzi, D.; Hassan, H. The influence of selenium supplementation on postpartum thyroid status in pregnant women with thyroid peroxidase autoantibodies. J. Clin. End. Metab. 2007, 92, 1263–1268. [CrossRef] [PubMed]
136. Rayman, M.P. Multiple nutritional factors and thyroid disease, with particular reference to autoimmune thyroid disease. Proc. Nutr. Soci. 2018, 1–11. [CrossRef] [PubMed]
137. Brigelius-Flohé, R.; Arnér, E.S. Selenium and selenoproteins in (redox) signaling, diseases, and animal models-200 year anniversary issue. Eur. PMC 2018, 127, 1–2. [CrossRef] [PubMed]
138. Wu,W.;Wang, Q.; Yin, F.; Yang, Z.; Zhang,W.; Gabra, H.; Li, L. Identification of proteomic and metabolic signatures associated with chemoresistance of human epithelial ovarian cancer. Int. J. Clin. Oncol. 2016, 49, 1651–1665. [CrossRef] [PubMed]
139. Agnani, D.; Camacho-Vanegas, O.; Camacho, C.; Lele, S.; Odunsi, K.; Cohen, S.; Dottino, P.; Martignetti, J.A. Decreased levels of serum glutathione peroxidase 3 are associated with papillary serous ovarian cancer and disease progression. J. Ovarian Res. 2011, 4, 18. [CrossRef] [PubMed]
140. Piekutowski, K.; Makarewicz, R.; Zachara, B. The antioxidative role of selenium in pathogenesis of cancer of the female reproductive system. Neoplasma 2007, 54, 374–378. [PubMed]
141. Falck, E.; Karlsson, S.; Carlsson, J.; Helenius, G.; Karlsson, M.; Klinga-Levan, K. Loss of glutathione peroxidase 3 expression is correlated with epigenetic mechanisms in endometrial adenocarcinoma. Cancer Cell Int. 2010, 10, 46. [CrossRef] [PubMed]
142. Saga, Y.; Ohwada, M.; Suzuki, M.; Konno, R.; Kigawa, J.; Ueno, S.; Mano, H. Glutathione peroxidase 3 is a candidate mechanism of anticancer drug resistance of ovarian clear cell adenocarcinoma. Oncol. Rep. 2008, 20, 1299–1303. [CrossRef] [PubMed]
143. Lee, H.J.; Do, J.H.; Bae, S.; Yang, S.; Zhang, X.; Lee, A.; Choi, Y.J.; Park, D.C.; Ahn,W.S. Immunohistochemical evidence for the over-expression of Glutathione peroxidase 3 in clear cell type ovarian adenocarcinoma. Med. Oncol. 2011, 28, 010–9659. [CrossRef] [PubMed]
144. Hough, C.D.; Cho, K.R.; Zonderman, A.B.; Schwartz, D.R.; Morin, P.J. Coordinately up-regulated genes in ovarian cancer. Cancer Res. 2001, 61, 3869–3876. [PubMed]
145. Rayman, M.P.; Winther, K.H.; Pastor-Barriuso, R.; Cold, F.; Thvilum, M.; Stranges, S.; Guallar, E.; Cold, S. Effect of long-term selenium supplementation on mortality: results from a multiple-dose, randomized controlled trial. Free Radical Biol. Med. 2018. [CrossRef] [PubMed]
本文由福山生物整理翻译,转载请注明出处。
上一篇:硒在甲状腺病理生理中的作用
下一篇:硒的预防癌症